Intelligent Design
Life Sciences
Encryption System Found in Genes
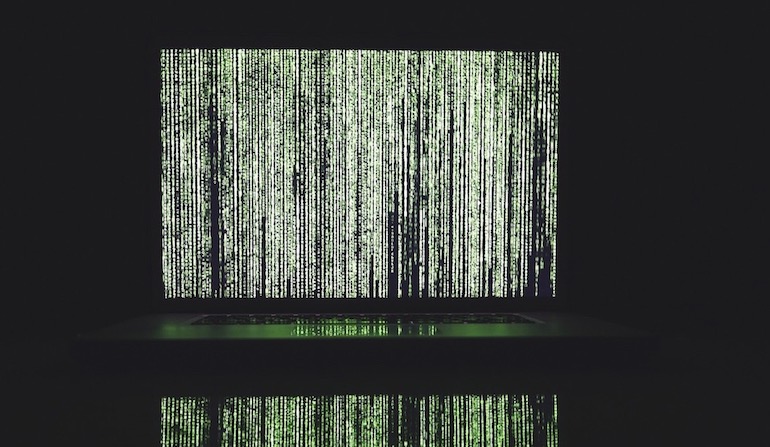
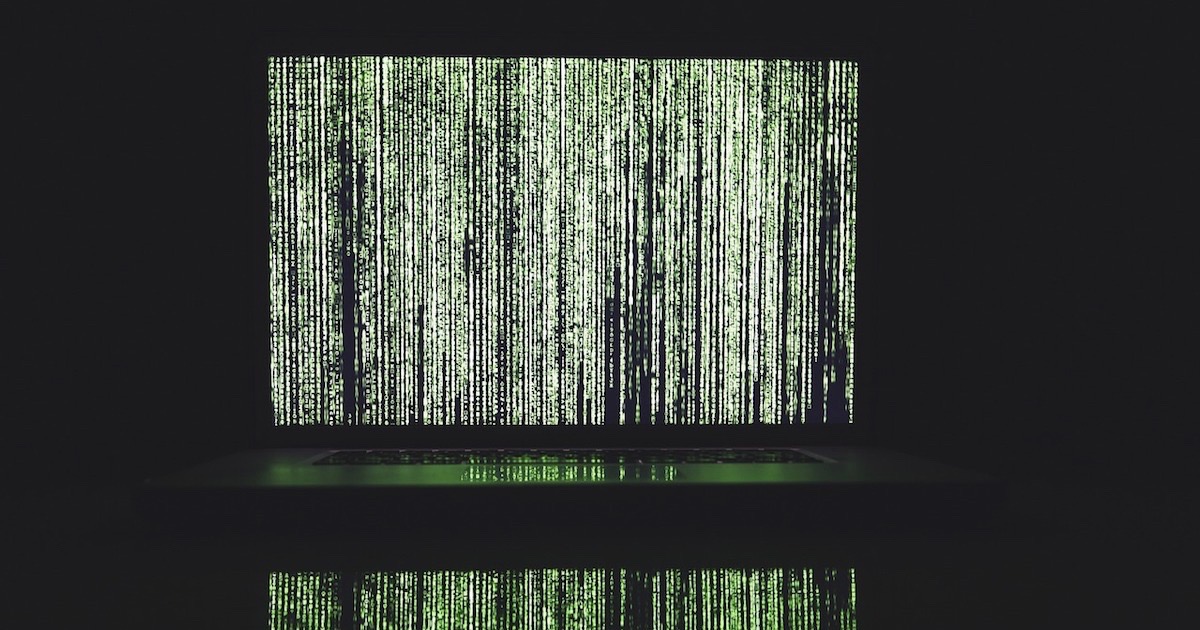
Researchers at Aarhus University in Denmark have uncovered a new form of gene regulation that appears to be a form of encryption of genetic information. That idea was not lost on them as they pursued the analogy. From “Encrypted messages in biological processes”:
RNA modifications can encrypt the RNA code and are responsible for a very sophisticated control of RNA function. A Danish-German research team has shown that modified RNA bases have a great impact on the dynamics of gene expression from DNA to functional RNA. The study yields important new insight into how the basis of RNA modifications can affect the function of mature RNA molecules. [Emphasis added.]
Programmers know all about cryptology, a form of intelligent design. Encryption is necessary when you want to conceal information from people who shouldn’t have access to it.
How to Achieve Encryption
There are many ways to achieve encryption, such as steganography, frame shifting, compression, and other techniques, but the function is to transfer a message in plain sight such that it can only be decrypted by those with the encryption “key.” Let’s learn how the cell does it.
RNA is composed of four bases (abbreviated A, U, G and C), thereby disseminating its message with a fairly simple code. In recent years, research has shown an unprecedented impact of RNA modifications at all steps of the maturation process. More than a hundred RNA modifications have been identified with roles in both inhibiting and facilitating binding to proteins, DNA and other RNA molecules. This encryption by RNA modification is a way to prevent the message of the RNA in being read by the wrong recipients.
The research-team has focused on the RNA-modification m6A and shown that RNA can be labeled with this modification while being copied from DNA…. The results demonstrate that an m6A positioned at an exon next to an intron increases the RNA maturation process, while m6A within the introns slows down the maturation of RNA (Figure 2).
For the encryption analogy to work, we need to see the algorithm targeting desired recipients and shielding the message from others. That’s evident from their statement that the “m6a” modification is able to “prevent the message of the RNA in being read by the wrong recipients.”
An Encryption Key
We also need to see an encryption key that can scramble the message, and a reader with the key that can unscramble it. Is this a possible role for the mysterious introns? The caption for Figure 2 explains:
Newly made RNA consists of functional parts (exons) and non-functional parts (introns). Introns are excised in a process called splicing to yield a mature and functional RNA molecule composes [sic] entirely of exons. The RNA modification m6A can increase or inhibit this maturation process dependent of where m6A is deposited on newly made RNA.
It has long been a mystery why genes code for stretches called introns that are translated but then cut out afterwards. Why are they there? Here’s where the findings get really interesting. Introns appear to help scramble the message, fulfilling the encryption role, but they do something else: they regulate how the exons will be assembled. The paper in Cell Reports explains how it works:
Here, we provide a time-resolved high-resolution assessment of m6A on nascent RNA transcripts and unveil its importance for the control of RNA splicing kinetics. We find that early co-transcriptional m6A deposition near splice junctions promotes fast splicing, while m6A modifications in introns are associated with long, slowly processed introns and alternative splicing events. In conclusion, we show that early m6A deposition specifies the fate of transcripts regarding splicing kinetics and alternative splicing.
We’ve heard about m6a before (January 2017) as a possible player in the “epigenetic code.” As an epigenetic marker, m6a contains its own information (the “key”) that affects the products of translation. A month later (February 2017) we learned that m6a “might have a role in alternative splicing of RNA transcripts,” which can yield many proteins from the same gene, depending on how the exons are rearranged. Last year, the findings were setting off a “gold rush” to uncover m6a’s function in the epigenetic code.
Rate of Production
Now, according to the scientists at Aarhus, the specific position of the m6a mark appears highly relevant not only to the type of messenger RNA produced — and thus the protein to be translated — but also to the rate of production. If the m6a mark is placed near a splice junction, the constitutive transcript is produced quickly (i.e., exons are arranged in the order they were transcribed). If the mark is placed on an intron, it slows down the splicing, and might produce a completely different transcript with a different protein resulting. Is this a method to achieve cell-specific regulation?
Our January 2017 article spoke of the m6a process as a kind of “if-then” algorithm: i.e., if this gene is found in a muscle cell, transcribe it this way; if found in a nerve cell, transcribe it another way, and so on. For this to work, the gene must embed the key in its introns, and the associated m6a marker must know the key to arrange the transcript accordingly. The researchers found that 57 percent of the markers were found on introns, and another 9 percent are on untranslated regions. Only 22 percent were found in coding regions.
Coming into Focus
It’s not just having the mark that directs the regulation. Previous research identified a “protein family called m6A readers, which recognize and bind specifically to sequences marked with m6A.” In addition, there are FTO pathways that respond to m6a position to either include or exclude certain exons in the transcript. We can see the encryption algorithm coming into focus: genes employ a key to embed introns and m6a markers, and certain readers that know the key respond according to this extra information.
The lack of strong consensus sequences at SJs [splice junctions] of many introns may be compensated by the presence of m6A that could eventually attract splicing factors to exert their function. Our study shows that the crucial role of m6A on SED [splicing efficiency dynamics] as well as on alternative splicing is position dependent. m6A deposited in intronic regions sort transcripts to a slow-track processing pathway and is associated with alternative splicing while m6A deposited at exonic boundaries of SJs sort transcripts to a fast-track processing pathway and constitutive splicing.
More work can be done to pursue this idea of encryption. For instance, what is the role of intron variability? The team found “Intron length and internal m6A signal are significantly associated with slow processing,” but is there additional information embedded in the introns beside length?
Design-focused research appears best poised to understand encryption algorithms and other information-rich systems. These researchers at Aarhus, who incidentally said nothing about evolution, seem to understand that.
Image credit: markusspiske, via Pixabay.