Intelligent Design
Life Sciences
Cell Machines Maintain the Planet for Life
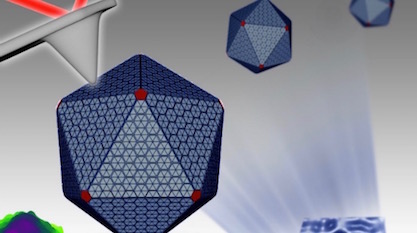
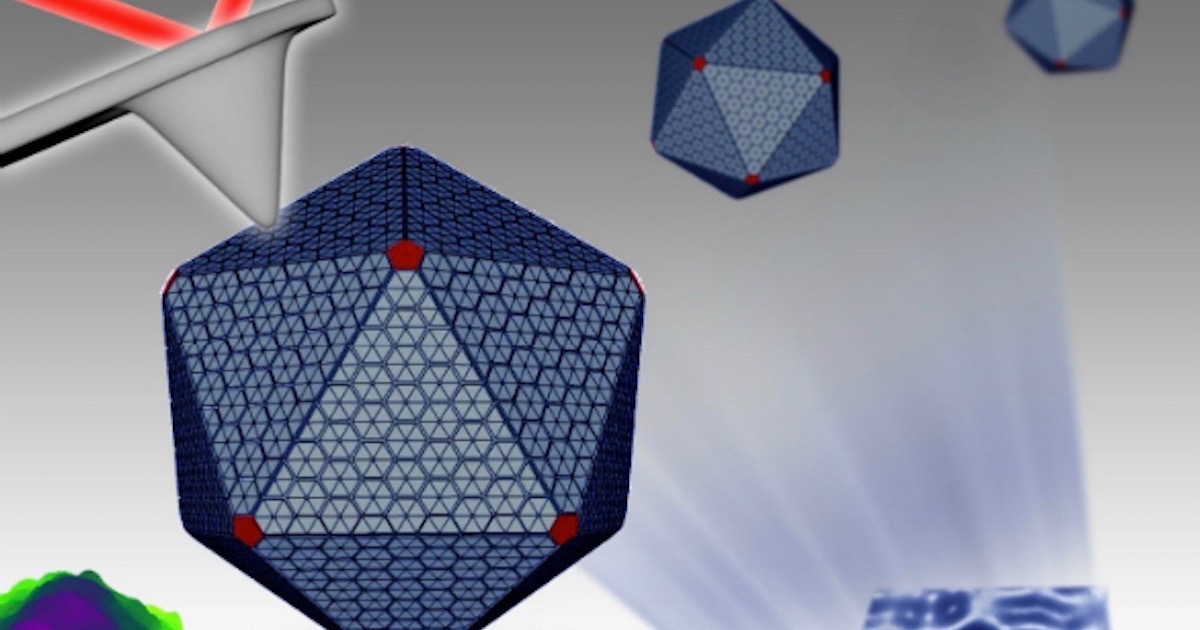
Count the mentions of the word “machines” in this news from the University of Liverpool:
- “Nanotechnology reveals hidden depths of bacterial ‘machines’…”
- “New research from the University of Liverpool, published in the journal Nanoscale, has probed the structure and material properties of protein machines in bacteria, which have the capacity to convert carbon dioxide into sugar through photosynthesis.”
- “Unique internal ‘machines’ in cyanobacteria, called carboxysomes, allow the organisms to convert carbon dioxide to sugar and provide impacts on global biomass production and our environment.”
- “So far, little is known about how these ‘machines’ are constructed and maintain their organisation to perform carbon fixation activity.”
- “They then used electron microscopy and atomic force microscopy to visualise the morphology and internal protein organization of these bacterial machines.”
- “‘We’re now just starting to understand how these bacterial machines are built and work in nature. Our long-term vision is to harness the knowledge to make further steps towards better design and engineering of bio-inspired machines,’ added Dr Liu. ‘The knowledge and techniques can be extended to other biological machines.’” [Emphasis added.]
That’s a lot of machine language for one short article! And it doesn’t even include related words like mechanical, nanotechnology, and structure. They’re talking about an amazing little organelle in cyanobacteria (once considered among the most primitive of cells) called the carboxysome — one of those tiny wonders most people don’t know about but depend on for life.
Cyanobacteria are a phylum of bacteria that produce oxygen and energy during photosynthesis, similar to green plants. They are among the most abundant organisms in oceans and fresh water.
As the article states, the machines in carboxysomes “provide impacts on global biomass production and our environment” by using sunlight to turn carbon dioxide into sugar, releasing oxygen for us to breathe.
Carboxysomes are polyhedral structures resembling a viral capsid, except that these living machine factories are “much softer and structurally flexible, which is correlated to their formation dynamics and regulation in bacteria.” Zooming in, we find higher levels of organization. The paper in Nanoscale says:
The protein shell serves as a physical barrier to protect enzymes from the cytosol and a selectively permeable membrane to mediate transport of enzyme substrates and products.
There’s more. The team found three structural domains inside, “a single-layered icosahedral shell, an inner layer and paracrystalline arrays of interior Rubisco.” Rubisco? Is that some kind of cracker? No — it’s another one of the most important things in nature you may have never heard of, and here it is, laid out in nice orderly rows in a geometric nanofactory.
Short for “Ribulose 1,5-bisphosphate carboxylase/oxygenase” (thank goodness), Rubisco is the most abundant protein on earth. According to PNAS, this machine solves an “abominably perplexing puzzle” of distinguishing between “featureless” molecules of carbon dioxide and oxygen, and it “may be nearly perfectly optimized” to do so. Once it was thought to be a slow, sluggish enzyme that leaks, but the authors find otherwise:
We assert that all Rubiscos may be nearly perfectly adapted to the differing CO2, O2, and thermal conditions in their subcellular environments, optimizing this compromise between CO2/O2 specificity and the maximum rate of catalytic turnover.
Commenting in Nature on that 2006 paper, Howard Griffiths added some amazing facts about Rubisco:
Rubisco has the reputation of being slow and inefficient, but it is one of life’s big successes: globally there is an estimated 5–10 kg Rubisco for every person on Earth, and each year it reacts with 15% of the total pool of atmospheric CO2.
Working alongside Rubisco in those neatly arranged layers inside the carboxysome is another amazing enzyme, carbonic anhydrase. Last year, we learned about how this “fine-tuned” enzyme (one of the few that employs zinc in its active site) helps salmon get an energy boost for their muscles when they leap waterfalls. Here it is again in cyanobacteria, working with Rubisco to regulate the levels of carbon dioxide by converting it to bicarbonate or back again as needed. The Protein Data Bank says, “Carbonic anhydrase is an enzyme that assists rapid inter-conversion of carbon dioxide and water into carbonic acid, protons and bicarbonate ions.” This ubiquitous enzyme is found in mammals, plants, algae, and bacteria.
The current study in Nanoscale explains why compartmentalization of these machines is important:
Within the cytosol which is a crowded and changing environment, it is important that carboxysomes are sufficiently robust to ensure the proper protein assembly, encapsulation of Rubisco enzymes and functional architecture. On the other hand, they are also flexible and dynamic to allow metabolite passage, turnover of building modules and interactions with other cellular components.
It’s not a prison, in other words, but a lively factory with windows and doors, yet it sequesters the machines safely for their protection. Carboxysomes are examples of bacterial microcompartments (BMCs) we’ve discussed before. These sure look designed: “The protein shell, structurally resembling virus capsids, is made of multiple protein paralogs forming hexagons and pentagons, and acts as a physical barrier that controls the passage of substrates and products of enzymatic reactions.” One can find spontaneous hexagons in nature, but some of them are clearly designed: those that exhibit “functional coherence” to use Doug Axe’s term (Undeniable, p. 160, pp. 178-181).
The authors don’t seem to have much use for evolutionary theory; anyway, they never mention it in the paper. But they did have design on their minds:
The study provides novel insights into the inherent structure and physical elasticity of native β-carboxysomes. It will empower our toolbox for the design and construction of functional metabolic machinery with applications in bioengineering and nanotechnology.
The report from the University of Liverpool amplifies the design theme:
“We’re now just starting to understand how these bacterial machines are built and work in nature. Our long-term vision is to harness the knowledge to make further steps towards better design and engineering of bio-inspired machines,” added Dr Liu, “The knowledge and techniques can be extended to other biological machines.”
Let’s end with some “wow” facts about a tiny cyanobacterium you may not have heard of. It contains these molecular factories (carboxysomes) loaded with Rubisco and carbonic anhydrase, working day and night to cleanse our atmosphere and regulate global carbon. Its name is Prochlorococcus. Sallie W. Chisholm says this about it in her Quick Guide in Current Biology:
- “We now know that there are about 100 million of these tiny powerhouses in each liter of seawater over vast oceanic regions,” and yet “they are 100 body lengths away” from each other.
- Prochlorococcus “is the smallest and most abundant photosynthetic cell on Earth.”
- “There is an estimated 3 x 10(exp)27 of them in the oceans, collectively weighing twice as much as all humans, and sporting a surface area 100 times that of the Earth.”
- “They constitute half of the chlorophyll over vast ocean ecosystems, single-handedly supplying significant amounts of organic carbon to the rest of the microbial food web.”
- “…they are extremely efficient photosynthetic machines.”
- “…their genomes represent one of the most streamlined blueprints for life.”
- “With a lower bound of 1,800 genes, they synthesize biomass using only solar energy, CO2 and inorganic compounds. That’s minimal life. That’s impressive!”
Carboxysomes rule the world. What if there had not been enough magnesium (for the chlorophyll) and zinc (for the carbonic anhydrase) close to the surface to supply the layers of enzymes in the factories of all those trillion quintillion cells? The Earth seems to have set the stage for complex life, a point Michael Denton would no doubt appreciate.
Image credit: University of Liverpool.