Evolution
Intelligent Design
No. 9 Story of 2023: Irreducible Complexity of Sperm Cells
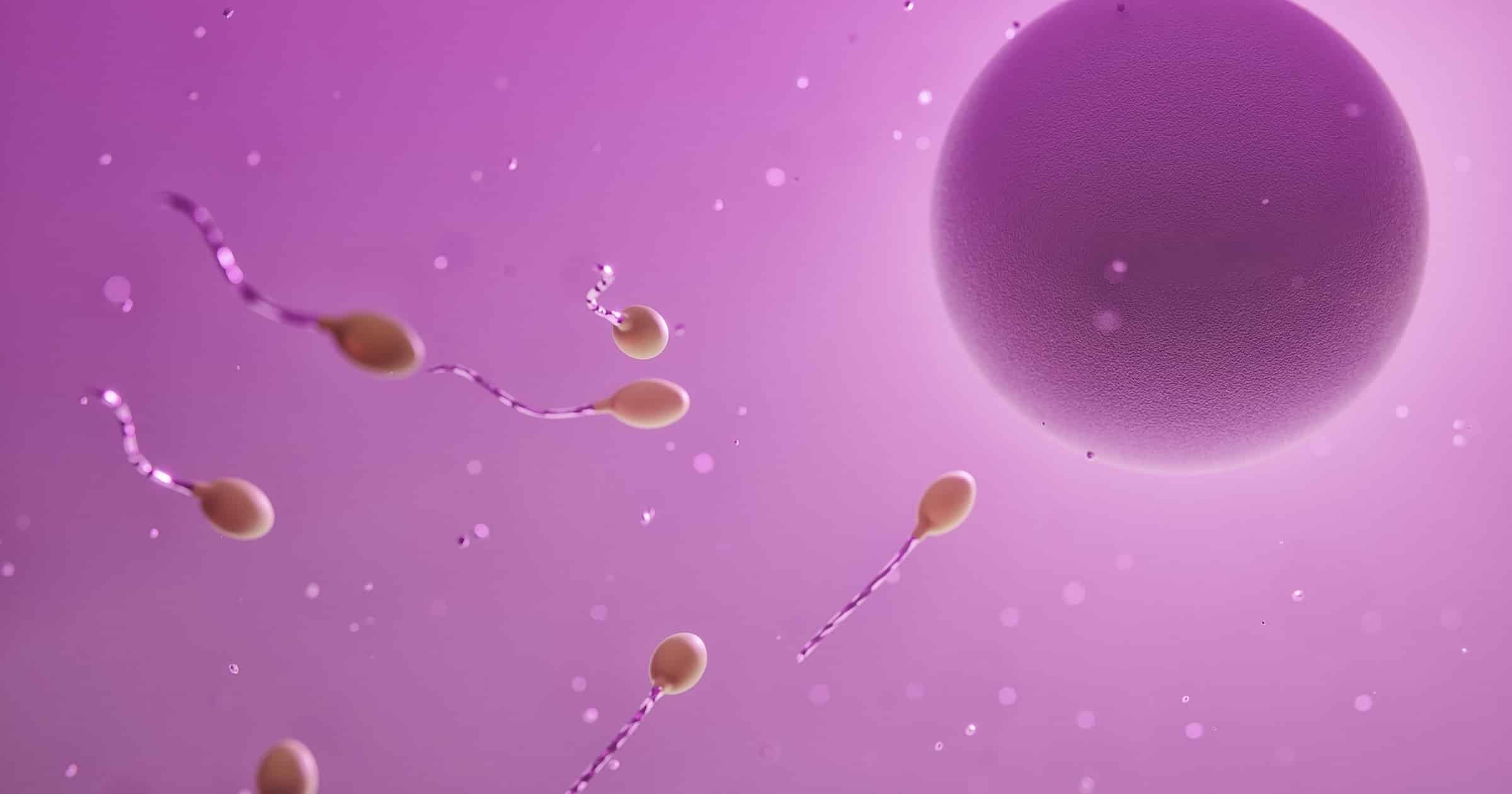
Editor’s note: Welcome to an Evolution News tradition: a countdown of our Top 10 favorite stories of the past year, concluding on New Year’s Day. Our staff are enjoying the holidays, as we hope that you are, too! Help keep the daily voice of intelligent design going strong. Please give whatever you can to support the Center for Science & Culture before the end of the year!
This article was originally published on June 30, 2023.
Human reproduction is perhaps the quintessential example of teleology in biology. The process by which a fertilized egg develops into an infant over the space of nine months reveals exquisite engineering and ingenious design. Before this intricate process can even begin, there is a need for a sperm cell to fuse with an ovum — each carrying, in the case of humans, 23 chromosomes. This incredible feat bears the unmistakable hallmarks of conscious intent and foresight. You can watch an animation of this remarkable process here:
Here, I will focus on the design characteristics of sperm cells. In a subsequent post, I will discuss the design features of the seminal fluid, and sperm capacitation. Sperm cells are comprised of three components — the head, the middle piece, and the flagellum — and hundreds of millions of them are carried in the seminal fluid that is released into the cervix through ejaculation during sexual intercourse. With each ejaculation, the male releases between two hundred and five hundred million sperm cells (approximately 100 million per milliliter of semen). Each of these three components, and the seminal fluid, is crucial to the sperm cell’s mission of fusing with an ovum to form a zygote (a fertilized egg). Let us consider each one in turn.
The Head
The head carries densely coiled chromatin fibers, containing the haploid genome — totaling half of the genetic material that will be inherited by the next generation (the other half will come from the mother’s egg cell). The tight packaging of the DNA serves to minimize its volume for transport.
On the tip of the sperm head is a membranous organelle, called the acrosome, that contains various hydrolytic enzymes. When these are secreted, they digest the egg cell membrane, thereby facilitating penetration of the ovum. Without the acrosome, the sperm cell will be unable to penetrate the egg cell membrane to fertilize the ovum. According to a review paper published in Frontiers in Cell and Developmental Biology:
Any structural or functional acrosomal abnormality could impair sperm fusion, and ultimately result in infertility. Moreover, studies have shown that intra-cytoplasmic insemination with sperm containing acrosomal abnormalities did not lead to successful fertilization, even in the absence of fertilization barriers, because the oocyte was unable to be efficiently activated…Thus, the acrosome is indispensable for fertilization.1
When a sperm reaches the vicinity of the egg, it undergoes a series of molecular interactions with the zona pellucida, which is a specialized extracellular matrix surrounding the egg. Specific receptors on the sperm’s plasma membrane, such as spermadhesins or integrins, recognize and bind to corresponding ligands on the zona pellucida. This binding triggers the activation of signaling pathways in the sperm. Binding of the sperm receptors to the zona pellucida ligands leads to an influx of calcium ions (Ca2+) into the sperm cell. This calcium influx is typically mediated by ion channels or receptors on the sperm’s plasma membrane, which are activated upon ligand-receptor binding. The increase in intracellular calcium levels initiates a signaling cascade within the sperm cell. Calcium ions act as second messengers and trigger the activation of various downstream signaling molecules and enzymes, including protein kinases. As a result of the calcium-mediated signaling cascade, the acrosome undergoes exocytosis. The membrane surrounding the acrosome fuses with the sperm’s plasma membrane, causing the release of the acrosomal contents, including enzymes such as hyaluronidase and acrosin. The enzymes released from the acrosome help degrade the glycoprotein matrix of the zona pellucida, allowing the sperm to penetrate and reach the egg’s plasma membrane. The acrosomal contents aid in the breakdown of the protective layers surrounding the egg, facilitating the fusion of the sperm and egg membranes.
The formation of the acrosome itself is divided into four stages. The first stage, the “Golgi phase,” is dependent upon the Golgi apparatus, which produces and packages the proteins and enzymes needed for acrosome formation. These proteins are then transported into the developing acrosome vesicle. In the second phase, the “cap phase,” the Golgi-derived vesicle (known as the proacrosomal vesicle) fuses with the anterior portion of the nucleus, forming a cap-like structure over the nucleus. The fusion of the vesicle with the nucleus is mediated by membrane trafficking processes. The proacrosomal vesicle contains enzymes, glycoproteins, and other components that are essential for acrosome maturation. In the third phase, the “acrosome phase,” the cap-like structure undergoes a series of structural changes, leading to the formation of the acrosome. The proacrosomal vesicle flattens and elongates, spreading over the anterior region of the nucleus. The Golgi-derived enzymes modify the proteins present in the proacrosomal vesicle, converting them into their active forms. The acrosomal membrane also undergoes changes, becoming specialized for the acrosome’s functions. In the final phase, the “maturation phase,” the acrosome undergoes further modifications and maturation. Enzymes within the acrosome become fully activated and the acrosomal matrix undergoes changes, becoming more condensed. The acrosomal granule, which is the central region of the acrosome, becomes highly electron-dense due to the accumulation of enzymes and proteins. The mature acrosome is now ready for its role in fertilization. For a more detailed description of this incredible process, I refer readers to a review paper on the “Mechanism of Acrosome Biogenesis in Mammals.”2
The Middle Piece
The middle piece consists of a central filamentous core, around which are many strategically placed mitochondria that synthesize the energy molecule adenosine triphosphate (ATP). The complexity and design of energy generation within the mitochondria — including the processes of glycolysis, the citric acid (or, Krebs) cycle, the electron transport chain, and oxidative phosphorylation — could be its own series of articles, but this is a topic for another day. For a good introduction to the phenomenal processes within the mitochondria, here are three animations from Harvard University that bring this fascinating organelle to life:
The ATP generated by the mitochondria energizes the power strokes of the flagellum, driving its journey through the female cervix, uterus, and uterine tubes. As such, the middle piece of the sperm cell is absolutely essential to its function of swimming through the female uterus and fallopian tube to fertilize her egg. Without the middle piece and its mitochondria, the sperm cells are completely immobile.
The Flagellum
Unlike a bacterial flagellum (which rotates like a motor), a sperm flagellum beats with a whip-like motion to produce motility. How does the flagellum work? In 2018, Jianfeng Lin and Daniela Nicastro elucidated the mechanism of flagellar motility.3 Their data indicated that “bending was generated by the asymmetric distribution of dynein activity on opposite sides of the flagellum”4 (dyneins are ATP-powered molecular motors that “walk” along microtubules towards their minus end). Their results also revealed that alternating flagellar bending occurs due to “a ‘switch-inhibition’ mechanism in which force imbalance is generated by inhibiting…dyneins on alternating sides of the flagellum.”5 In other words, regulatory signals lead to the inhibition of dynein motors on one side of the flagellum. Meanwhile, on the other side, the dyneins walk along the microtubules. The flagellum bends in one direction due to molecular linkers that resist this sliding. The flagellar bending alternates by repeatedly switching the side of dynein inhibition. Look here for an animation showing how this is thought to work.
It goes without saying that, without the flagellum, the sperm cell is completely immotile and has no chance of fertilizing the egg.
Thus far, we have considered the irreducible complexity of the components of a sperm cell. In a subsequent article, we shall consider the design features of the seminal fluid and the process of sperm capacitation that takes place within the female reproductive tract.
Notes
- Khawar MB, Gao H, Li W. Mechanism of Acrosome Biogenesis in Mammals. Front Cell Dev Biol. 2019 Sep 18;7:195.
- Ibid.
- Lin J, Nicastro D. Asymmetric distribution and spatial switching of dynein activity generates ciliary motility. Science. 2018 Apr 27;360(6387):eaar1968.
- Ibid.
- Ibid.