Intelligent Design
Physical Sciences
The Remarkable Carbon Atom
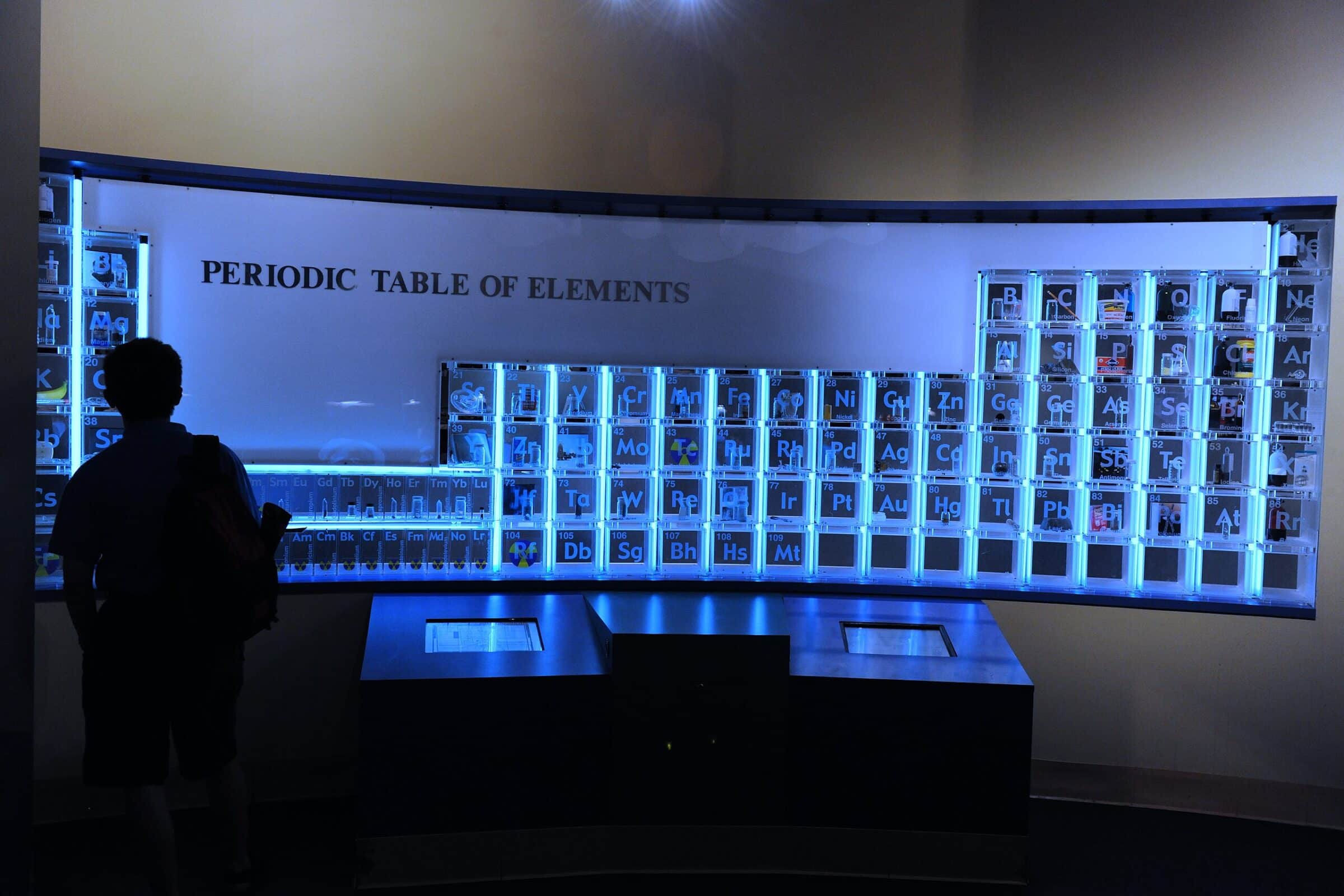
In an article yesterday, I discussed the incredible design of the nonmetal atoms, and the striking coincidence that the very atoms from which one can build stable, defined shapes also give us the hydrophobic force, which is the key to arranging them into higher-level structures. Here, I will discuss the fitness of carbon for life, and the incredibly fortuitous circumstances that promote its abundance in the universe.
The Fitness of Carbon for Life
The carbon atom, the primary constituent of organic molecules, is, in several respects, uniquely fit for the assembly of the complex macromolecules found in the cell. First, due to the stability of carbon-carbon bonding, only carbon can form long polymers of itself, forming long chains or rings, while also bonding to other kinds of atoms. Though silicon can also form long chains by bonding with itself, these bonds are significantly less stable than carbon-carbon bonds. Plaxco and Gross note that “while silicon-silicon, silicon-hydrogen, and silicon-nitrogen bonds are similar in energy, the silicon-oxygen bond is far more stable than any of the other three types. As a consequence, silicon readily oxidizes to silicon dioxide, limiting the chemistry available to this atom whenever oxygen is present. And oxygen is the third most common atom in the Universe.”1 As Primo Levi explains, carbon “is the only element that can bind itself in long stable chains without a great expense of energy, and for life on earth (the only one we know so far) precisely long chains are required. Therefore carbon is the key element of living substance.”2
Second, carbon is tetravalent — that is, each atom can form four covalent bonds with other atoms. Third, carbon possesses a relatively small atomic nucleus, entailing short bond distances, thereby allowing it to form stable bonds with itself as well as other atoms. This property is also possessed by the other small, non-metal atoms in period two. Carbon is able to form single, double, and triple bonds with other atoms. Nitrogen can also form single, double, or triple bonds and oxygen can form single and double bonds. Contrast this with the nonmetal atoms directly beneath them in the periodic table — silicon, phosphorus, and sulfur — which possess larger atomic radii and therefore form such bonds less easily due to multiple bonds having reduced stability.
Another property of organic bonds is that their strength sits within a Goldilocks zone, being neither too strong nor too weak for biochemical manipulations in the cell. If the strength of those bonds were to be altered by a single order of magnitude, it would render impossible numerous biochemical reactions that take place in the cell. If it were too strong, the activation energy needed to break bonds could not be sufficiently reduced by enzymatic activity (enzymes strain chemical bonds by engaging in specific conformational movements while bound to a substrate). Conversely, if organic bonds were much weaker, bonds would be frequently disrupted by molecular collisions, rendering controlled chemistry impossible.
Another special characteristic of carbon is that there is not much variation in energy levels of carbon bonds from one atom to the next. Robert E. D. Clark explains that carbon “is a friend of all. Its bond energies with hydrogen, chlorine, nitrogen, oxygen, or even another carbon differ little. No other atom is like it.”3 Kevin W. Plaxco and Michael Gross further comment, “Carbon presents a fairly level playing field in which nature can shuffle around carbon-carbon, carbon-nitrogen, and carbon-oxygen single and double bonds without playing too great a cost to convert any one of these into another… Given all this, it’s no wonder that on the order of ten million unique carbon compounds have been described by chemists, which is as many as all of the described non-carbon-containing compounds put together.”4
Carbon Resonance
As we have seen, carbon is absolutely fundamental to life. It also happens to be — after hydrogen, helium, and oxygen — the fourth most abundant element in our galaxy. A carbon nucleus can be generated by smashing together two nuclei of helium-4 to make beryllium-8 (containing four protons and four neutrons) and then adding a further nucleus of helium to generate carbon-12 (containing six protons and six neutrons). However, beryllium is quite unstable, and can be expected to break apart into two nuclei of helium in 10-16seconds. On occasion, prior to the breaking apart of beryllium, a third helium nucleus collides with beryllium, resulting in a carbon nucleus. As it happens, the carbon atom possesses a special quantum property called a resonance, which facilitates this process. A resonance describes the discrete energy levels at which protons and neutrons in the nucleus can exist. Indeed, it turns out that the resonance of the carbon atom just so happens to correspond to the combined energy of the beryllium atom and a colliding nucleus of helium.
As Geraint Lewis and Luke Barnes explain, “if there were a resonance at just the right place in carbon, the combined energy of the beryllium and helium nuclei would result in a carbon nucleus in one of its excited states. The excited carbon nucleus knows how to handle the excess energy without simply falling apart. It is less likely to disintegrate, and more likely to decay to the ground state with the emission of a gamma-ray photon. Carbon formed, energy released… success!”5 Without this specific resonance level, the universe would contain relatively few carbon atoms — in 1953, this specific resonance that had previously been predicted by Fred Hoyle was discovered by William Fowler, precisely where Hoyle had predicted it would be.
A Remarkable Coincidence
This special carbon resonance (known as the Hoyle state), which corresponds to the energy levels of the combined beryllium-8 nucleus and a helium-4 nucleus, renders the otherwise improbable process of carbon-12 formation feasible and efficient in the high-temperature environments of stellar cores. This delicate balance of energy levels is a remarkable aspect of nuclear astrophysics that allows for the creation of the elements necessary for life. If it were not for this special resonance, life very probably would not exist in our universe. This is another one of many countless features of our universe that have to be “just right” for life — in particular, advanced life — to exist.
Notes
- Kevin W. Plaxco and Michael Gross. Astrobiology: A Brief Introduction, 2nd edition (The John Hopkins University Press, 2011), chapter 1.
- Primo Levi, The Periodic Table (Abacus, 1990), 226-227.
- Robert E.D. Clark, The Universe: Plan or Accident? 3rd edition, (Zondervan, 1972), 97.
- Kevin W. Plaxco and Michael Gross. Astrobiology: A Brief Introduction, 2nd edition (The John Hopkins University Press, 2011), chapter 1.
- Geraint F. Lewis and Luke A. Barnes, A Fortunate Universe: Life in a Finely Tuned Cosmos (Cambridge University Press, 2017), 116-117.