Intelligent Design
Life Sciences
Tendons Are Irreducibly Complex
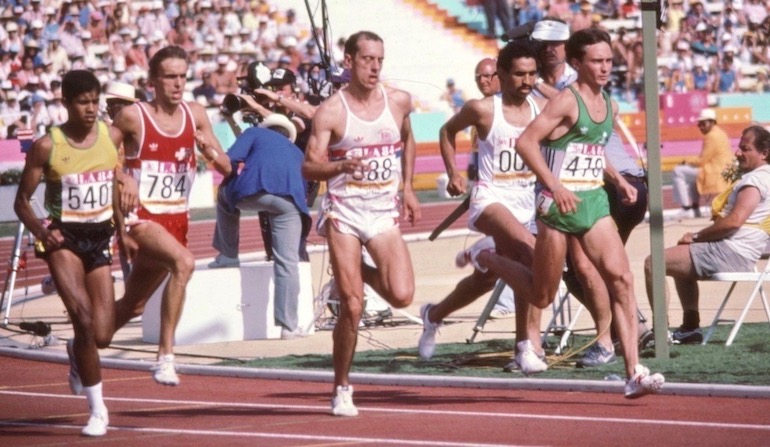
The tendons that hold your skeleton together are diverse, tightly knit, and overlapping in complex ways. The optimization of tendons for function extends from the macroscale to the nanoscale.
Anatomists have understood the look and feel of tendons ever since the early days of dissection, but only recently have scientists been able to study their nanostructure. A new paper in Nature’s open-access journal Scientific Reports reveals that “In tendons, differing physiological requirements lead to functionally distinct nanostructures.” They begin with a summary of what tendons do:
While collectively referred to as tendons, the physiological functions served by the tissues that connect muscle to bone vary considerably within certain animals, including humans. Tendons like the digital extensors and flexors of the hand transmit forces in such a way that fingers can be moved with great precision. Other tendons, like the Achilles, function as springs that enable locomotive activities such as running and jumping to be performed efficiently by storing energy during deceleration, and then releasing it to help power acceleration. [Emphasis added.]
Already we’re learning amazing things about tendons to earn our respect. In his series “The Designed Body” in these pages two years ago, Howard Glicksman described how tendons are attached to muscles so as to handle tension and prevent strain.
Next, the researchers talk physiology. How do tendons function in activities of life? Some tendons function just by virtue of their position. Others are highly dynamic, acting as load-bearing springs. Notice how robust and resilient tendons are, able to perform repetitive actions many times over a lifetime of basketball, walking, or playing a piano. Marathon runners should pay attention to this paragraph:
Tendons that store and release energy face differing functional demands to those that are primarily positional in nature. Unlike positional tendons, energy storing tendons must be able to withstand large forces applied in a highly repetitive manner. When running, for example, tension in the Achilles tendon during ground contact exceeds 12 times body weight; in the case of a marathon, the tendon must endure this loading about 25,000 times without rest. In other tissues such as bone, demanding mechanical loading regimes are dealt with via remodeling, where fatigue damage occurs, but its excessive accumulation is prevented by turnover of the damaged components. Surprisingly, despite undergoing high stress cyclic loading, energy storing tendons do not undergo appreciable remodeling. Rather than continually repair accrued damage, these specialized tendons appear to have evolved highly fatigue resistant structures.
Whether “tendons appear to have evolved” their surprising characteristics is a question for later. (Incidentally, this is the only mention of evolution in the paper.) At the nanoscale, however, tendons become even more amazing.
The collagen-based tissues of animals are hierarchical structures: even tendon, the simplest collagenous tissue, has seven to eight levels of hierarchy. Tailoring tissue structure to match physiological function can occur at many different levels. We wanted to know if the control of tissue architecture to achieve function extends down to the nanoscale level of the individual, cable-like collagen fibrils….
With their differing functions in mind, we wanted to determine if energy storing and positional tendons were composed of functionally distinct collagen fibrils. Collagen fibrils are very fine, precision built, biological cables, each millimeters long but only a hundred nanometers or so in diameter. Fibrils gain tensile strength via intermolecular crosslinking, which transmits load from one triple-helical collagen molecule to the next within the fibril structure. Tendons are composed of millions of these collagen fibrils packed in parallel, which together make up about three-quarters of a young, healthy tendon’s dry weight.
Some Canadian’s lunch hamburger served up some science on the side. For the research, the authors extracted tendons from steers slaughtered for food in Nova Scotia. The scientists took the collagen fibrils and tested their strength to the point of rupture, their resistance to unraveling, and the options for remodeling.
Using tendons from young adult bovine forelimbs, we performed stress-strain experiments on single collagen fibrils extracted from tendons with positional function, and tendons with energy storing function. Collagen fibrils from the two tendon types, which have known differences in intermolecular crosslinking, showed numerous differences in their responses to elongation. Unlike those from positional tendons, fibrils from energy storing tendons showed high strain stiffening and resistance to disruption in both molecular packing and conformation, helping to explain how these high stress tissues withstand millions of loading cycles with little reparative remodeling. Functional differences in load-bearing tissues are accompanied by important differences in nanoscale collagen fibril structure.
Millions of loading cycles! What human engineering could hope to match the tendon’s specifications for decades? Think of every step you take, every object you manipulate with fingers, every bending or stretching exercise. Most of us accomplish such things easily for many decades. And think of the range of loads these tissues can endure, from the lightest touch on a keyboard to a weightlifter’s 200-kg clean and jerk. Every basketball player, high jumper or marathoner puts incredible stress on tendons, far beyond what is necessary for mere survival.
In conclusion, the research team learned that positional and energy-storing tendons, having different requirements for repair, rely on the way the fibrils are organized at the molecular level.
Collagen fibrils from energy storing tendons are clearly structurally and mechanically different to those making up positional tendons. The presence of trivalent crosslinks within energy storing fibrils appears to limit molecular sliding, making the fibril structure resistant to permanent and irrecoverable unwinding of the collagen triple-helix. The ability to retain normal structure under severe loading conditions may explain the ability of energy storing tendons to function with little reparative cellular remodeling during a person’s lifetime. An open question remains: what advantage do positional tendons gain by having collagen fibrils that are susceptible to significant structural disruption when overloaded? The presence of trivalent crosslinking has been linked to a decreased enzymatic susceptibility of collagen fibrils. It may be that frequent turnover of collagen fibrils in positional tendons, and thus limitation of advanced glycation endproduct crosslink accumulation, is important to the tendons’ proper physiologic function, and that susceptibility to severe mechanical disruption is a necessary trade-off for a fibrillar structure that facilitates remodeling.
Thinking like design engineers, the authors detect reasons for the ways the fibrils are wrapped and organized. Trade-offs are compromises made to optimize the highest design goal. In a laptop computer, for instance, light weight and battery life require trade-offs in disk size or screen dimensions. A “necessary trade-off” implies a design constraint. In positional tendons, we see a possible reason for susceptibility to mechanical disruption that would be catastrophic for energy-storing tendons. The body provides a workaround for the trade-off by creating a structure that facilitates repair and remodeling. Energy storing tendons cannot afford to be “down” as long, and so have a more robust “normal structure” at the nanoscale.
We’ve seen seven to eight levels of hierarchy in a tendon’s structure — enough to begin thinking about irreducible complexity (IC). Add to that the physical constraints of ability to withstand rupture while storing energy, the crosslinks, and the repair options, and the case for IC grows. It becomes overwhelmingly persuasive when we consider that the fibers are coded by genes and built by ribosomes using two independent codes: the genetic code and the protein code. On top of that, consider that the fibrils must be sent to the proper location in the body and wrapped with others at the proper angle by other molecular machines.
All this is done “blind” by molecules without eyes and brains, as if they were programmed by a master designer to function for a lifetime without the user’s conscious thought. As if they were? They were.