Intelligent Design
Welcome to the Electric Cell
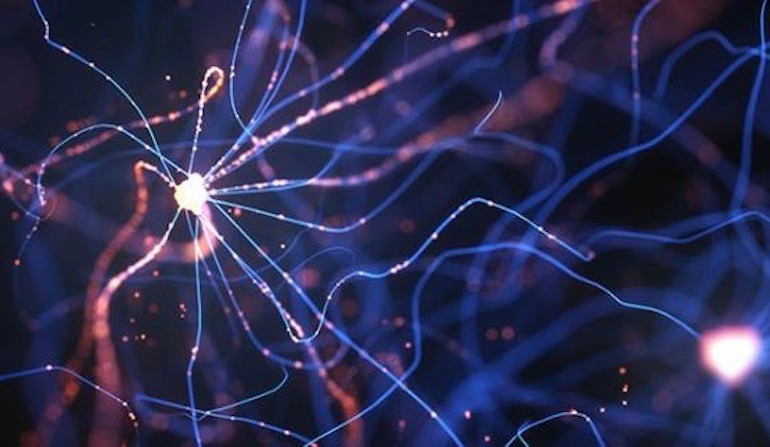
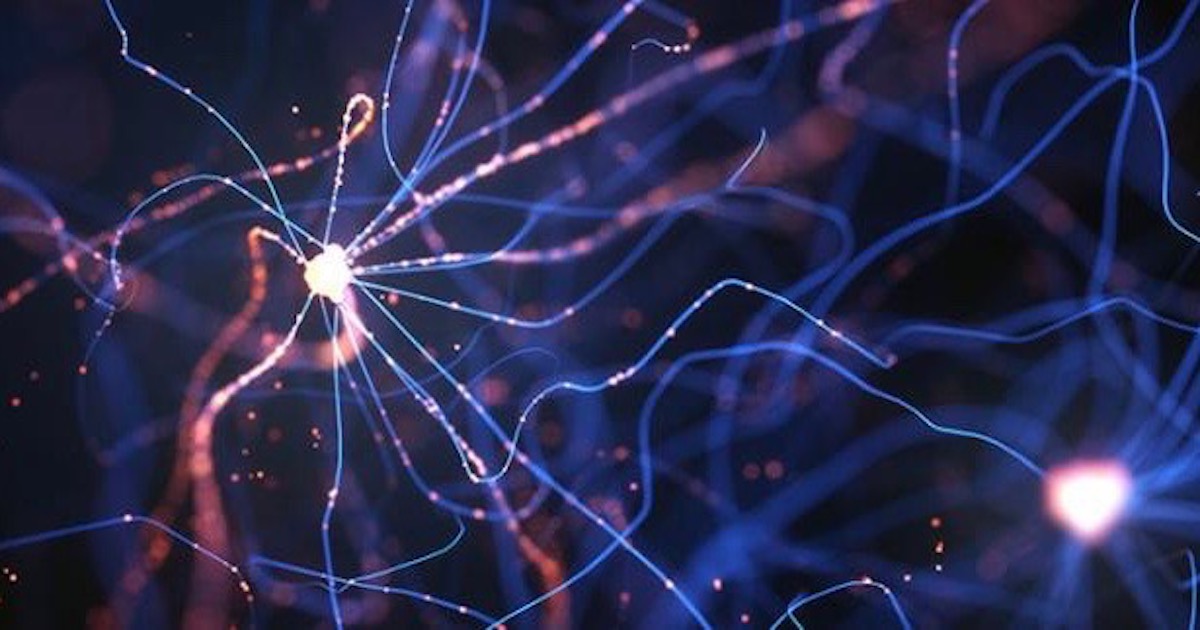
Neurons have well-known quasi-electrical processes. Specialized membrane channels admit positively-charged potassium ions and sodium ions into the cytoplasm, and pump them out, creating pulses that travel down axons or dendrites. Synapses convert the pulses to chemical signals, and back again. Neurologists speak of “action potentials” as these ion flows show up as electrical spikes in the inner ear, the olfactory organs, and other parts of the central nervous system. Other examples include the engines ATP synthase and bacterial flagella, which rotate by means of motive forces produced by protons or sodium ions.
These processes, while employing charged particles, look more like chemistry than electricity. Now, however, some scientists are describing real electrical currents passing through “wires” of a kind.
The Electric Cell
Our modern electrical grids transmit more than power. They also transmit information. The words and pictures on our screens come via electromagnetic (EM) pulses that become the 1’s and 0’s computers recognize. Some pulses, also called solitons, come through the air in wireless transmissions of EM waves via cell towers, satellites or WiFi routers in our homes. Other informational pulses pass through coaxial cables or power lines (“broadband over power lines,” or BPL). Are cells wired for electrical transmission of information? Indeed, they are. A paper by B. R. Frieden and R. A. Gatenby in Nature Scientific Reports says this possibility is backed up by evidence:
Although the elements of the cytoskeleton are primarily involved in cellular shape and movement, they can also serve as both biomechanical and electrical conduits of information that can alter gene expression and chromosomal location. The ability of microfilaments and microtubules to conduct electrons and ions has been extensively documented. Microfilaments are actin polymers which maintain high levels of negative surface charges permitting highly dynamical interactions with both within the microfilament and in exchange with cytoplasmic counterions. A number of studies have demonstrated charge centers with corresponding counter ion clouds along the microfilament axis permitting ionic waves propagating along its long axis. This conductance takes on a specific form as Cantiello et al. demonstrated actin filaments propagate electrical signal via soliton waves so that the signal is virtually lossless. [Emphasis added.]
The possibility of electrical transmission exists, therefore, but does it really happen? What kind of information is conducted down the cell’s transmission lines?
There is also experimental evidence that microtubules can regulate VDAC ion channels [voltage-dependent ion channels within membranes] in the mitochondria and that microtubules can influence and be influenced by the extracellular matrix (ECM), so that there is a dynamic and ongoing exchange of information between the cytoskeleton and cell exterior.
Awesome Vistas for ID
This opens up awesome new investigations for intelligent design, both on the nature of the information transmitted, and on the infrastructure used to transmit it. Consider that the transmission lines of actin and microtubule filaments, being constructed of protein building blocks, are an order of magnitude smaller than the neurons that conduct action potentials along their length. This newly acknowledged electrical network overlays the well-known signal transduction system that transmits information in the form of chemicals packaged in vesicles of clathrin or other coat proteins (see “Cell Vesicles Wear Sophisticated Coats”). The Electric Cell concept expands on 2015’s announcement that mitochondria are linked in a cellular power grid (see “Spectacular Discovery Reveals Power Grid in Muscle Cells; Design Implications Are Profound”). Frieden and Gatenby say:
Hence we investigate the potential of microtubules and microfilaments to act as information conductors that link the cell membrane with central cellular structures including the nucleus, mitochondria and endoplasmic reticulum. These thereby form a distributed information network of conductors.
Many Questions Can Now Be Investigated
How is the information coded and decoded? Can the code be deciphered? How do receptors transduce the information into forms they understand? How does this electrical information system interact with the chemical information system? How do the nanowires avoid short circuits? What are the voltage, amperage and resistance values? Readers of this open-access paper will find delicious discussions of interest from a design perspective:
The dynamics governing information transmission have been extensively investigated by the pioneering work of Fisher, Shannon and others (see below). Ideal information transmission occurs when the receiver obtains precisely the information that was sent from its source. This is because, any channel carrying a signal from a sender to a receiver cannot (by definition of a channel) convey more information than is contained in the source signal. Instead, there is inevitable loss of source Shannon information en route during the process of encoding, transmission, reception and decoding of the message. Therefore, minimizing such loss is the realistic goal of such a system.
Note that this ignores the evolutionary cost to the system of acquiring the message. Instead, it tacitly assumes every such possible message to be acquired with equal cost, and focuses upon the issue of how well the system can respond to that message, regardless of cost….
It will be no surprise to ID advocates that cells are already well-optimized for information transmission:
In the case of microtubules, which typically converge on the centrosome, this coarse grain information allows rapid assessment of the overall state of the environment over time. Or in the case of microfilaments, which typically link to protein complexes on the nuclear membrane, the fine-grain information can convey, to the nucleus, detailed information about the spatial and temporal variations of the environment. In prior work we found these information dynamics to be highly optimized.
In other words, the cell is optimized to utilize both lossy information and detailed information, getting the best mix for rapid response. (A similar mix can be seen in DNA repair mechanisms, some of which are fast and error-prone, and others that are slow and careful.) Furthermore, both lossy and detailed information channels interact with each other and with other cellular signaling systems:
Finally, we note that our analysis ignores possible communication between individual microfilaments and microtubules. In reality, microfilaments and microtubules frequently interface through direct physical contact and cross-linking proteins. It is also likely that the elements of the cytoskeleton can interact in complex way with molecular transduction pathways. This suggests a complex network for signal transmission and analysis that permits rich information dynamics that likely augments and modifies the more well-known and studied information found molecular pathways (e.g. the MAPK pathway) that carry information following ligand binding to a membrane receptor to the nucleus.
New Research Opportunities
To see how these findings are already motivating research, look how Marcelo Marucho at the University of Texas at San Antonio is getting involved. News from the university says, “Now biophysics research at The University of Texas at San Antonio (UTSA) has taken a closer look at a cell’s cytoskeleton and found a new purpose: It aids in energy transfer and information processing in neurons.”
“There is evidence that filaments conduct electricity, like an electrical cord,” said Marucho, who combines both biology and physics to understand what he calls the bionanowire — a cell’s electrical cable. “The filaments, F-Actin and microtubules, are able to propagate electrical impulses inside neurons. This property is something that scientists generally haven’t considered.”
The cell’s cytoskeleton, or filaments, are responsible for a cell’s shape. They also organize cellular parts and impact a cell’s movement and division. But Marucho believes that cells cytoskeleton filaments are also capable of conducting electrical energy.
Marucho has received $1.5 million in grants from the NIH for his work. He is already conducting experiments “to determine how electricity spreads and how it affects the mechanical properties of filaments.” The potential for ID-based experiments on information transfer through bioelectricity within the cell appears to be wide open. Here is a great opportunity to take the lead, reap the implications, and even help mankind by identifying solutions for breakdowns that lead to disease.
Photo credit: University of Texas at San Antonio, via EurekAlert!