Intelligent Design
Life Sciences
Some Proteins Act Almost Like Humans
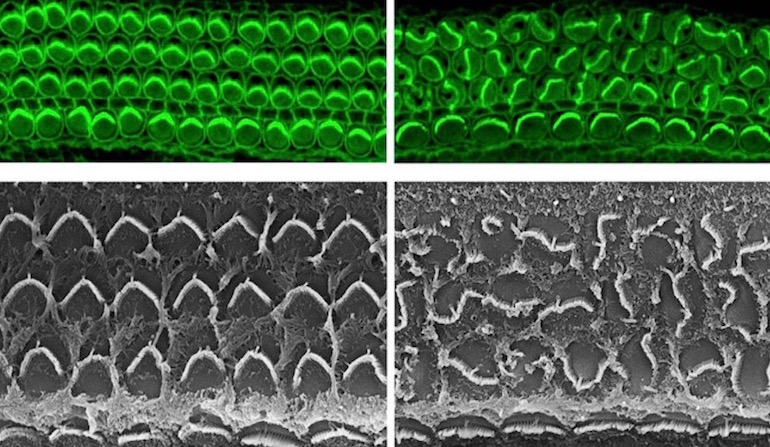
If you watched a well-designed humanoid robot like Star Trek’s Lieutenant Commander Data, you might be excused for thinking it (he?) was a real human. (Of course, Brent Spiner, the actor who played him, is.) With the right programming, a machine could pass the Turing test, fooling an observer into attributing sentience to it. But we don’t have to wait centuries for engineering to catch up with Star Trek. In our own bodies, there are proteins that get pretty close.
The Drum Major
Meet Daple, a protein in the inner ear. Those of us whose ears work properly can thank Daple for doing a good job when we were developing in the womb. Deep inside the cochlea of our inner ear, the robot-like Daple was guiding the construction of hair cell bundles, those important antennas that pick up fluid motions and transduce them into electrical impulses in the auditory nerve. Arranged like organ pipes, each hair cell bundle must line up properly to function. The individual hair cells have an assistant protein that puts them in their correct relative position, and another set of proteins controls the axis they need to line up on, but what brings these two functions together?
Until now, scientists did not know how these independent processes were coordinated. We can imagine the confusion of a marching band at half time without a drum major. Who directs the trumpets to go left and the tubas to go right? Who signals when things need to happen? The drum major has been found, a new paper reports in the Proceedings of the National Academy of Sciences (PNAS), and its name is — you guessed it — Daple. News from the Jackson Laboratory explains, how the band “concert” ends up playing on schedule and in tune:
…each individual hair cell must develop a motion sensor in the form of a brush of protrusions, or hair bundle, that is precisely organized and shows directionality, like the magnetized needle of a compass. In addition, neighboring hair cells also orient their bundles in concert, the same way a collection of compasses would all point to the north magnetic pole.
Both single-cell and organ-wide directionality are critical for hearing, but these two levels of organization are controlled by largely distinct molecular mechanisms during inner ear development. “Planar cell polarity” proteins establish a north and south pole in the organ, while the Gpsm2/LGN-Gai proteins act in single hair cells to help give the bundle directionality. How the two systems are reconciled for normal sensory function remained a mystery so far, however.
Now, a collaboration between a research team at The Jackson Laboratory (JAX) and The Rockefeller University has identified a protein, Daple, that interacts with both systems and is a promising candidate to coordinate single cell and organ-wide directionality. [Emphasis added.]
You can see from the electron micrographs (at the top of this post) that hair bundles without Daple are as disorganized as a band scattered on the field without a leader. For coordinating all the parts so that the whole formation comes together on time and in tune, providing its user with up to a century or more of musical enjoyment, we nominate Daple for Drum Major of the Year.
While the paper says nothing about evolution, the description at Science Daily does so in the familiar gratuitous manner:
Humans inherited the capacity to hear sounds thanks to structures that evolved millions of years ago. Sensory “hair cells” in the inner ear have the amazing ability to convert sound waves into electrical signals and transmit them to the brain for processing.
The Wisdom of the Crowd
Skin is a marvelous thing. It grows with us, never tearing, and (until we age) never sagging. Always renewing itself, it is our biggest organ. How does it “know” not to become too taut, making us struggle as if we were wearing too-tight clothing, or too loose, like trying to work in baggy pants? The Max Planck Institute asks that question:
Adult skin epidermis is build [sic] of different layers. Stem cells reside in the bottom layer where their task is to produce new cells which then differentiate and move upwards into the more specialized upper layer. This differentiation process involves permanent changes in the cell’s properties to best suit to serve skin’s barrier function. The skin must maintain balanced numbers of stem and differentiated cells as loss of proper balance would result in aberrant tissue structure and therefore function. How this intricate balance is maintained remained largely unknown until recently.
Lead author Yekaterina Miroshnikova describes what she calls “self-organization” behind this balance of cell types, but it looks more like collective wisdom:
“We observed that dividing stem cells induced a local crowding effect to the stem cell layer which deformed the cells in the vicinity of this event. Intriguingly, this compression and deformation triggered the differentiation of the neighboring cell”, explains Miroshnikova. The crowded and squeezed cells change their properties, leading to their ‘escape’ from the local stress in the bottom layer and upward movement. “The fact that cells sense what their neighbors are doing and do the exact opposite provides a very efficient and simple way to maintain tissue size, architecture and function”, says Miroshnikova.
It would be good if our own human neighbors had such good sense.
Showing Up for Work
Cabbies know to congregate at airports. Day laborers know to congregate at visible intersections. And proteasomes know to congregate where their skill is likely to be required. Proteasomes? Those are the molecular machines that disassemble spent proteins. We have described these shredders as multi-component machines with moving parts. Now, German researchers writing in PNAS find that they tend to congregate at two distinct sites, as if they had cab drivers to take them there. “Where to today, mister?” “The NPC terminal, please.”
Nuclear proteasomes were tethered to two different sites at the nuclear pore complex (NPC): the inner nuclear membrane and the NPC basket. Structural analysis revealed mechanistic details of the two tethering interactions. These results present direct evidence that proteasomes bind at NPCs, establishing a cellular hub for protein degradation at the gateway between the nucleus and cytoplasm.
Not every cab driver works at the airport, and not every day laborer meets at the same intersection. Similarly, proteasomes can exist anywhere inside or outside the nucleus. Somehow, large numbers of them meet at the NPC, as if they “know” that’s where they are likely to be gainfully employed. The open-access paper says:
These basket-tethered and membrane-tethered proteasomes, which have similar substrate-processing state frequencies as proteasomes elsewhere in the cell, are ideally positioned to regulate transcription and perform quality control of both soluble and membrane proteins transiting the NPC.
Conclusion
Each of these proteins operates in the dark, jostling interior of a cell, and yet has an uncanny sense of what it needs to do and where it needs to be. Each one cooperates with other proteins, sometimes coordinating the actions of large numbers of them. Daple never hears the band concert. Adult stem cells never feel a hot shower. Proteasomes never taste a holiday dinner. Without a brain or a mind to guide them, these machines work as if they have a higher purpose that calls for their skills, even though that purpose transcends their own microscopic existence. The effect is the health of an entirely different entity that is orders of magnitude larger than the protein — the human body.
It takes phenomenal programming to make a machine imitate a person. These molecular machines, silently fulfilling their coded instructions, come pretty close to passing the Turing test.
Photo credit: Electron micrographs via Jackson Laboratory.