Evolution
Intelligent Design
Morphogenesis: Coding for Shape
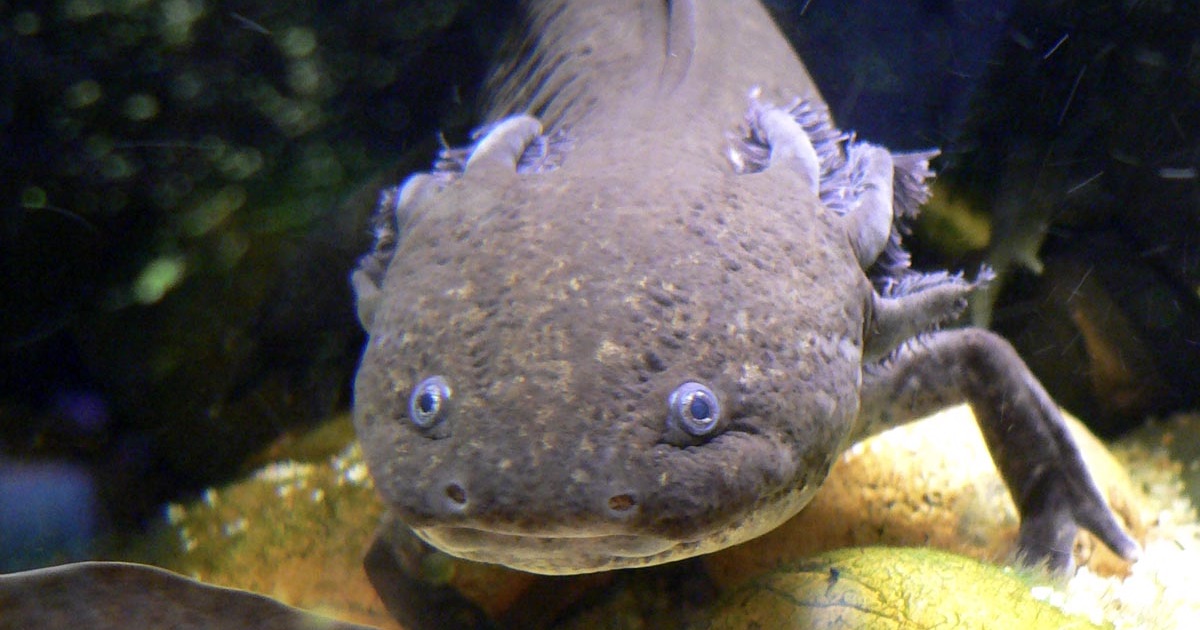
Organisms are hierarchies of shapes. Bacteria form rods, spirals, and spheres. Single-celled eukaryotes build diverse organelles inside and take on a characteristic shape outside (compare Stentor, Paramecium, and Amoeba). Think of all the varieties of shapes in multicellular organisms from Volvox to complex eukaryotes — hydra, rotifers, planaria at the microscopic end; crabs, octopuses, and beetles in the lower middle range; beavers, roses, and humans at the upper middle range; whales, sequoias, and brachiosaurs at the large end. Plants generate stems, leaves, and flowers. Animals grow tissues that organize into organs that combine into body plans. How do all these 3-D shapes emerge from a linear code? That is the puzzle of morphogenesis.
Functional Wholes
Biologists know about genetic codes for molecules fairly well now, but where is the software for anatomy? The recent fad for 3-D printing is crude by comparison. Those machines can produce a shape from a linear code, but they simply build a static object a layer at a time using homogeneous material. Morphogenesis requires bringing together diverse materials to construct moving machines, like hearts. These must continue functioning at all levels while being networked with other moving machines during construction. The end product is what Douglas Axe calls a “functional whole” (Undeniable, p. 143). Functional wholes in biology are composed of hierarchically organized functioning components and sub-components and elementary constituents that not only work in three-dimensional space, but over the fourth dimension of time. They possess the remarkable property of self-repair, too.
Michael Levin, director of the Allen Discovery Center at Tufts University and Associate Faculty at Harvard University’s Wyss Institute, is baffled by the origin of biological shapes. He writes in The Scientist:
While genomes predictably encode the proteins present in cells, a simple molecular parts list does not tell us enough about the anatomical layout or regenerative potential of the body that the cells will work to construct. Genomes are not a blueprint for anatomy, and genome editing is fundamentally limited by the fact that it’s very hard to infer which genes to tweak, and how, to achieve desired complex anatomical outcomes. Similarly, stem cells generate the building blocks of organs, but the ability to organize specific cell types into a working human hand or eye has been and will be beyond the grasp of direct manipulation for a very long time. [Emphasis added.]
In the movie Terminator 2, the assassin from the future is smashed into a thousand fragments of liquid metal, and then reconstitutes itself to carry on its mission. It is a very clever piece of special effects, but when you think about the problem, how could each fragment know where to go? And yet something like that happens in organisms that are able to regenerate themselves, like hydras, planarians, axolotls, and a few other species. Something like that also occurs during embryonic development. After multiple rounds of cell division of clones, diversification begins, and shape begins to emerge. Each cell gains a role and a destination in which to fulfill that role. See Illustra Media’s video clip about chick embryonic development:
Beyond DNA
In human embryonic development, something beyond DNA tells the growing mass how many liver cells are needed, how they should organize into the familiar liver shape, how many blood vessels are needed to supply the liver. In addition, something regulates how these shapes coordinate their growth from baby to adult. The liver always ends up at the proper size and position under the ribs on the right side, with the right connections to other organs. All the organs and systems follow this goal-directed process. Achieving this outcome requires much more information than the DNA code possesses for liver enzymes alone, as complex as that is. Where is “biology’s software — the rules that enable great plasticity in how cell collectives generate reliable anatomies”?
Answering the question will require interdisciplinary research, Levin points out. Scientists have only taken baby steps to tackle this enormous puzzle. All they can do currently is try to break down the question into manageable sub-questions.
But researchers working in the fields of synthetic morphology and regenerative biophysics are beginning to understand the rules governing the plasticity of organ growth and repair. Rather than micromanaging tasks that are too complex to implement directly at the cellular or molecular level, what if we solved the mystery of how groups of cells cooperate to construct specific multicellular bodies during embryogenesis and regeneration? Perhaps then we could figure out how to motivate cell collectives to build whatever anatomical features we want.
So far, they have only gotten frog embryos to grow weird synthetic shapes by genetic engineering. It’s an exciting start, Levin thinks, but the work resembles children in a playground.
These cells rebooted their multicellularity into a new form, without genomic changes. This represents an extremely exciting sandbox in which bioengineers can play, with the aim of decoding the logic of anatomical and behavioral control, as well as understanding the plasticity of cells and the relationship of genomes to anatomies.
A Biological Revolution
This work could represent the start of a biological revolution as significant as the genomic revolution when genetics graduated from molecules to codes. It represents the next step up: “elucidating the computations that cells and groups of cells carry out to orchestrate tissue and organ construction on a whole-body scale.” It’s as if biochemists have gotten a grip on how musical instruments are made, and now want to see how the music is performed, and how music is derived from a score coded by silent symbols on a page. But they’re trying to do all this without the central player: the composer!
The next generation of advances in this area of research will emerge from the flow of ideas between computer scientists and biologists. Unlocking the full potential of regenerative medicine will require biology to take the journey computer science has already taken, from focusing on the hardware — the proteins and biochemical pathways that carry out cellular operations — to the physiological software that enables networks of cells to acquire, store, and act on information about organ and indeed whole-body geometry.
In the computer world, this transition from rewiring hardware to reprogramming the information flow by changing the inputs gave rise to the information technology revolution. This shift of perspective could transform biology, allowing scientists to achieve the still-futuristic visions of regenerative medicine.
The effort can also transform engineering, he says. Engineers can learn from how organisms build structures that still work in noisy environments and can remain robust to perturbations. Even when his group genetically engineers frog embryos, Levin says, the embryos tend to find their way back to the target shape, as if a monitoring process constantly compares its activities with the ideal shape. How is that information stored and communicated?
The remarkable thing is not simply that growth begins after wounding and that various cell types are generated, but that these bodies will grow and remodel until a correct anatomy is complete, and then they stop. How does the system identify the correct target morphology, orchestrate individual cell behaviors to get there, and determine when the job is done? How does it communicate this information to control underlying cell activities?
Those are stimulating questions for the ID community to consider. ID understands foresight, control, and communication.
Is Darwinism Up to the Task?
Levin tries to bring evolution into the picture.
Evolution exploits three modalities to achieve such anatomical homeostasis: biochemical gradients, bioelectric circuits, and biophysical forces. These interact to enable the same large-scale form to arise despite significant perturbations.
But that is not Darwinian evolution at all! A mindless, aimless physical process doesn’t care what happens. It cannot exploit. It cannot achieve. It cannot enable. This statement is like putting a Darwin sticker on intelligently designed machinery. Much less can Darwinism signal, create, and operate electrical networks, make decisions, or regulate anything.
Observing is not explaining. Levin’s group can watch what happens, but he appeals to causes that are inadequate to account for them. The team can tweak working processes to achieve modified outcomes, but not explain their emergence. They can imitate them, but not originate them. They can compare them to intelligently designed computers and software, but not account for the similarities by appealing to opposite causes.
The emerging picture in this field is that anatomical software is highly modular — a key property that computer scientists exploit as subroutines and that most likely contributes in large part to biological evolvability and evolutionary plasticity.
“Evolvability” and “evolutionary plasticity” are highly misleading terms. What Levin means is the capability to learn and adapt to circumstances. That takes design. And why must plasticity be evolutionary? Take the e-word out and recognize the concept as robustness. That’s also design. Tolerance to perturbations, with some leeway for change, is a good design strategy. Musical compositions allow for some plasticity, too, as when the composer marks “Ad lib” for an improvisation or allows room for a cadenza. Works can be modified for different ensembles, too, like when an orchestral work is transcribed for chamber orchestra or piano.
Here’s another example of affixing a “Made by Darwin” sticker on design concepts:
In molecular biomedicine, we are still focused largely on manipulating the cellular hardware — the proteins that each cell can exploit. But evolution has ensured that cellular collectives use this versatile machinery to process information flexibly and implement a wide range of large-scale body shape outcomes. This is biology’s software: the memory, plasticity, and reprogrammability of morphogenetic control networks.
Such a claim makes no sense. Evolution cannot understand machinery or ensure that cells use it.
Is Design Science Up to the Task?
Only design science has the conceptual framework to understand this “new kind of epigenetics, information that is stored in a medium other than DNA sequences and chromatin.” Information Technology (IT) is design science by definition. Levin essentially repeats Darwin’s fallacy of using artificial selection as an analogue of natural selection, except that in morphogenesis, we infer the activity of a designing intelligence from its effects and from our uniform experience with a mind’s ability to organize components to reach targets reliably.
Will progress slow if ID takes the lead in morphogenesis research? Certainly not. Design scientists can continue the work on frog embryos and genetic engineering. In fact, they will likely work more productively unencumbered by the baggage of Darwin’s hoary Victorian myth.
Chance is not a cause; intelligence is. Intelligence can conceive of a plan, exercise foresight to identify the requirements, and then execute the plan by programming the components to fulfill the plan. At the forefront of this major biological revolution, it’s time to recognize that anatomical software is intelligent design all the way down.