Evolution
Intelligent Design
Noise Cancellation: A Remarkable Design Solution in Biology
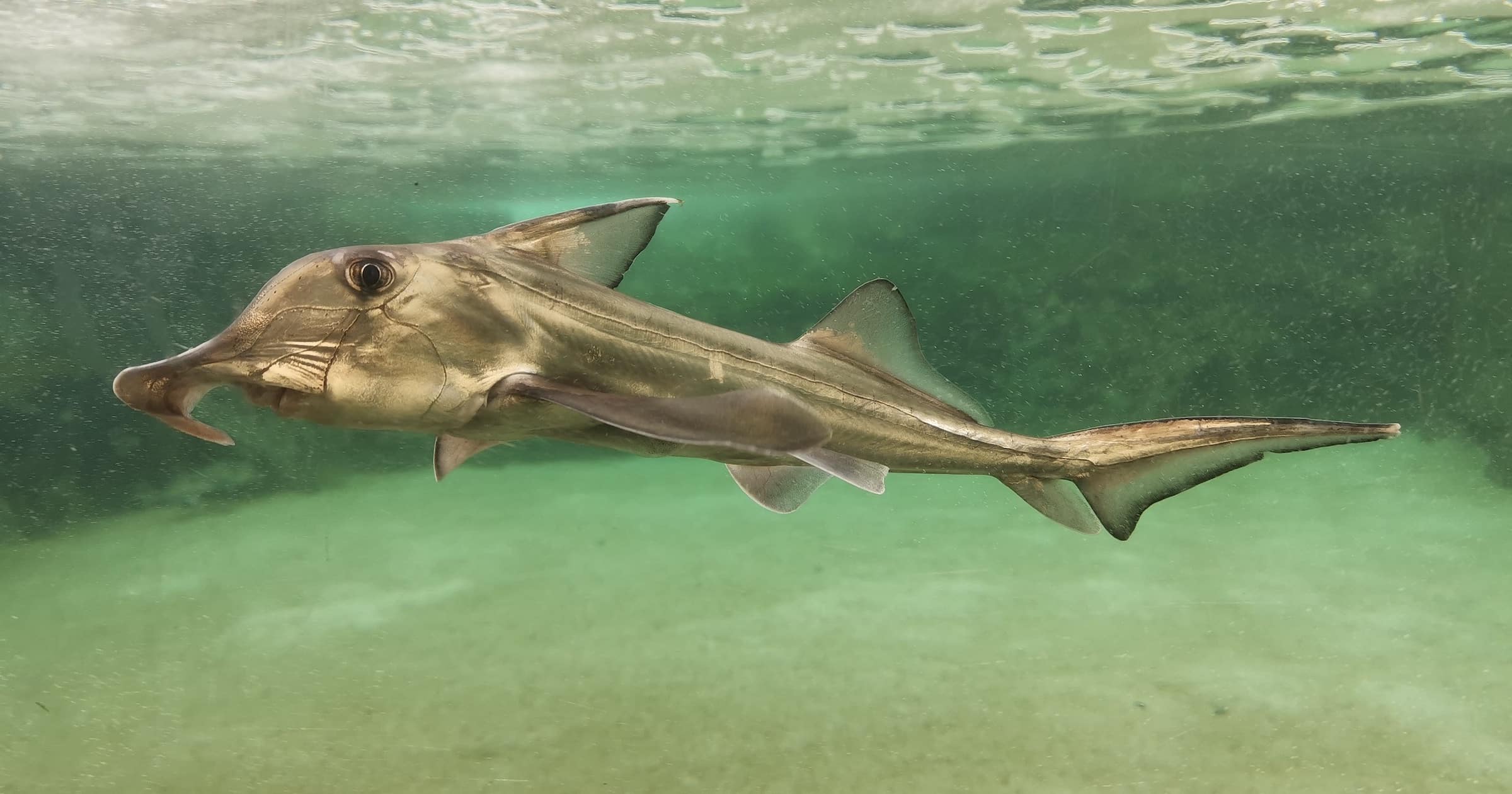
Snakes should be immune to their own poison. Electric eels should not shock themselves. And protection from self-generated noise requires a preplanned noise cancellation system.
In a Dispatch in Current Biology, Leonard Maier discussed a biological requirement many don’t think much about: how to ignore your own noise. Eliminating self-generated noise, he says, is accomplished by “Active Sensing.”
Animals use active sensing to investigate their environment. The active sense inputs must be discriminated from those arising independently from environmental signals. An experimental and modelling study has revealed how precise control of dendritic spike backpropagation contributes to such discrimination. [Emphasis added.]
The study referenced by Maier was published in Current Biology by Muller, Abbott, and Sawtelle. It involves some heavy reading in neuroscience, but the basic idea is easy to understand. If you’re trying to listen to something while making loud noises yourself, you need a way to subtract your own noise.
Biological noise cancellation works at the neuron level. The basic idea of “spike backpropagation” is that the receiving neuron sends precisely controlled signals to the sending neuron with an “image” (so to speak) of its own noise profile. This negative image cancels the noise part of the complete signal, eliminating self-generated noise from the received signal, so that the brain receives only the environmental signal.
By analogy, consider how the James Webb Space Telescope images extremely faint objects in the infrared part of the spectrum. The telescope and its instruments generate heat that would swamp any photons from the target object. One method is to cool the instruments down as far as possible to reduce self-generated heat. This is done with liquid helium. Another method is to subtract spectral lines generated by the telescope.
Noise cancellation is also used by exoplanet searches, as done by the Kepler mission (2009-2018). The spectral signature of a transiting planet would normally be overwhelmed by the brightness of its host star. Astronomers subtract the star’s light before and after the transit to detect the planet. The orbiting solar observatory SOHO used a similar method with its coronagraph, creating an artificial eclipse that blocked the bright light of the sun so that the faint corona could be observed. Noise cancellation is performed visually in adaptive optics and photographic noise reduction, and audibly with Dolby noise reduction and noise-cancelling headphones. The latter case is noteworthy for listening to sounds outside and inside the earphone, then inverting them to neutralize the outside sounds. One tech site says it is “A bit like taking +2 outside and adding -2 inside to make zero.”
Each of these techniques requires foresight and engineering precision to pull off. Additionally, the methods require continuous tracking (active sensing) to keep the instruments aligned properly and responsive to changing conditions.
Noise-Cancelling Fish
To find that biology uses noise cancellation is both surprising and logical. An organism’s sensors must be able to differentiate between self and non-self. Muller et al. made their discovery about signal backpropagation by experimenting on mormyrid fish. These are weakly electric fish that can generate currents as well as passively receive them. The “elephantfish” is an example of a mormyrid.
Neuroscientists have found mormyrids useful for studying biological signal processing, because the fish sends out electric pulses that need to be attenuated by the brain, and receives signals from conspecifics and from prey. Maier explains why a noise cancellation system had to exist. Sadly, he gives credit to evolution for a system that acts with “surgical precision” —
The electrosense is comprised of passive and active electroreception. The passive electrosense (ampullary receptors) is highly sensitive to the weak electric fields generated by movements of invertebrate prey. Activeelectroreception requires the generation of a brief (∼1 ms) electric organ discharge by an electric organ, and tuberous receptors tuned to the electric field produced by the electric organ discharge. Mormyrid fish have retained the passive sense and evolved the active electrosense essential for spatial learning and navigation that makes locating prey mor efficient. The evolution of the active sense comes at a price for mormyrid fish (specifically Gnathoneumus petersii). The brief mormyrid electric organ discharge evokes a large ringing spiking response in ampullary receptors that lasts ∼200 ms. The electric organ discharge rate ranges from 5 Hz (rest) to 60 Hz (foraging) and the ringing response overlaps the prey related sensory input. Ampullary receptors should be swamped by this noise and identification of prey obliterated. Ampullary receptors project to output cells within the medullary electrosensory lobe. Remarkably, electrosensory lobe output neurons respond faithfully to passive electrosensory input with no hint of their cacophonous input. There must be a mechanism that eliminates the ringing noise with surgical precision.
The fish’s method is just like noise cancellation in the technologies mentioned: subtract the unwanted noise to get the signal.
Cancellation of ringing noise is via learning a ‘negative image’ equal and opposite to the ringing response, followed by summation of the ringing noise and its negative image. The key to the negative image lies in a predictive corollary discharge signal that precisely times the occurrence of each electric organ discharge. The corollary discharge reaches cerebellar granule cells which, in turn, project to apical dendrites of the medium ganglion cells. The medium ganglion cells learn to precisely follow the ringing noise and their inhibitory input to the output cells summates with and cancels their ringing noise input.
It’s a clever trick, but even more remarkable in the details. The neurons along the signal path have to adjust for the propagation rate and know when to potentiate (increase) the spikes or depress (decrease) them to create the “negative image.” Width, amplitude, and timing of the spikes are crucial to success. This image (actually a backward-propagating pulse train) must be faithfully reproduced across synapses, where electrical signals are converted to chemical signals and vice versa. In addition, the precision extends to the types of ion channels (e.g., sodium or calcium) in the axons and dendrites. It’s mind boggling to read the details in Maier’s synopsis and Muller et al.’s research. But there’s more: hormones are involved, too.
Signal Prioritization
Another paper about noise cancellation in mormyrid fish appeared in Current Biology a month after the Muller paper. Fukutoni and Carlson determined that the system also relies on hormones — in particular, testosterone — to coordinate the motor output and internal prediction of sensory consequences. In breeding males, they found, “inhibition activated by a corollary discharge blocks sensory responses to self-generated electric pulses, allowing the downstream circuit to selectively analyze communication signals from nearby fish” to determine if they are juveniles, females, or non-reproductive males. “In this case, testosterone directly affects the biophysical properties of the electrocytes in the electric organ that determine the EOD [electric organ discharge] waveform.” If evolution had not thought of that set of random mutations needing selection, then the poor fish would have gone extinct. (The authors didn’t say that; just trying to be logical.)
Regarding this twist on the subject, Washington University in St. Louis took note that a “hormone alters electric fish’s signal-canceling trick.” It boils down to timing control.
The electric fish known as mormyrids send out electric pulses as signals; they also have developed a way to ignore or block their own messages. A system called corollary discharge inhibits the fish’s sensory perception for a brief, well-defined period of time after it releases an electric pulse — allowing it to prioritize messages from others, such as potential mates.
Other Cases in Biology
What is revealed in these papers is a remarkably effective noise cancellation technology in fish. They can sense faint electrical signals from prey and from conspecifics despite initiating electrical pulses into the water 60 times a second! Those pulses would swamp the faint signal from the target, were it not for a combination of neurons, ion channels, mathematically precise algorithms and hormones working together to subtract the self-signal and hone the target signal. The resultant signal, furthermore, must activate instinctive behaviors or it would accomplish nothing.
The principle behind this biological methodology can be extended to every case in biology where an organism, whether a cell or a fruit fly or a grizzly bear, needs to discriminate signals from self to respond to environmental signals. Grizzly bears, I have heard, give off a very strong odor. Yet their sense of smell is remarkably sensitive. They must be able to perform “smell cancellation” to subtract their own odor from the signal they wish to focus on. Echolocation wouldn’t work if the dolphin or bat was unable to discriminate between its own clicks and the echoes from the target. You can think up more examples, and perhaps curiosity about how they are achieved will generate some fruitful research projects for advocates of intelligent design.