Evolution
Intelligent Design
Origin of Life: The Challenge of Achieving Homochirality with Mineral Surfaces
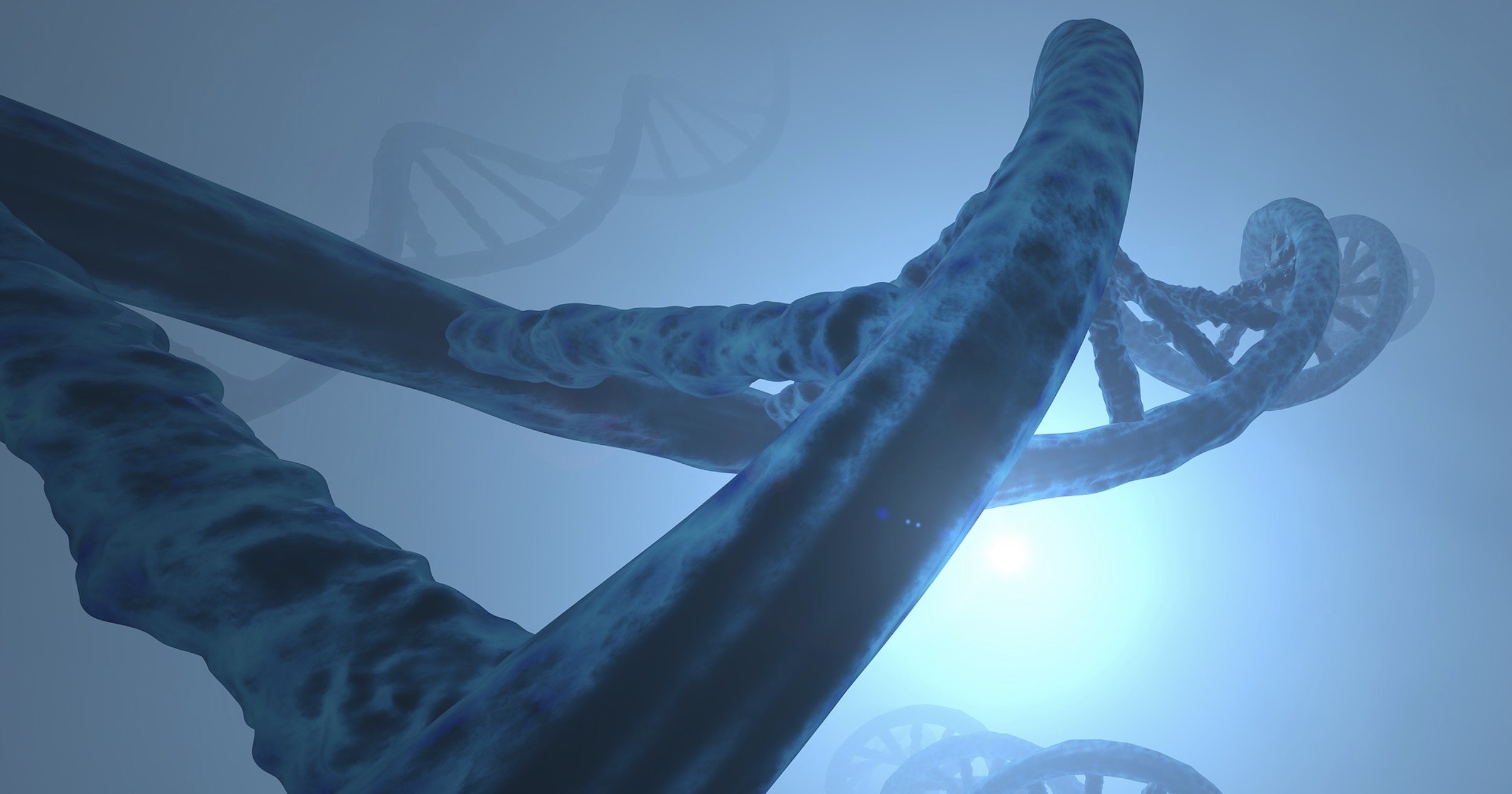
It’s a challenge crucial for research on the origin of life. Among the many obstacles to generating biologically relevant polymers abiotically, achieving homochirality is perhaps the most difficult impediment to overcome. What is homochirality? Well, all macromolecules in life are composed of building blocks that exist as mirror images. The analogy of left and right hands is most commonly used to convey this chemical concept. With very few exceptions, life only uses one of these forms.
The chemical properties of mirror imaged compounds are equivalent for all practical purposes. The production of these building blocks in a prebiotic world, using very simple compounds as starting material, would therefore be expected to result in a racemic mixture, or 50/50 distribution, of these building blocks. This has already been confirmed from analysis of organic compounds retrieved from an asteroid, as I wrote here back in May.
Producing polymers consisting of only one configuration from this mixture is obligatory if a prebiotic origin of life on Earth is to be convincingly explained to the scientific community. Proposing chemical schemes that produce polypeptides (proteins) or RNA using homochiral building blocks is the task at hand. Performing such a feat in solution appears unfeasible, so the prebiotic soup approach has been abandoned by many origin of life (OOL) scientists. A better alternative, widely investigated, is the use of mineral surfaces where adsorption of organic chemicals could theoretically select preferentially one configuration of a pair of chiral molecules.
Here I will briefly discuss this experimental approach, noting where the relevant studies have led. I’ll first consider polymerization of amino acids to form proteins, followed by attempts to achieve this feat using RNA precursors.
From Racemic Amino Acids to Homochiral Proteins
Among studies showing efficacy in obtaining short lengths of amino acids to form oligopeptides, one of earliest was reported in 1978 (Lahav N., White D., and Chang S. (1978) Science 201: 67-69). The system employed, to facilitate the condensation reaction to form peptide bonds between amino acids, was the use of clay minerals (kaolinite and bentonite). Repeated cycles of heating, evaporation, and rehydration were invoked, which are conditions likely present on a prebiotic Earth. Using the simplest amino acid, glycine (having no chiral center), diglycine was found to be produced at low levels with progressively lower amounts up to pentapeptide lengths. Notably the heating-drying phase of this cycle apparently facilitates the condensation reaction, enabling peptide bond formation.
A Long Way from the Goal
This was a start, but still a long way from producing polypeptide lengths of any biological relevance. Small proteins should reach at a minimum 100-200 amino acids to effectively contribute to biological function. After this initial glimmer of hope, many other laboratories undertook similar approaches, testing out different mineral surfaces and a variety of reaction conditions. The highest achievers managed to produce polypeptides up to decamers. It became clear from these studies that the longer the polypeptide produced, the harder it was to retrieve it from the mineral surface, as it was tightly bound via a multitude of chemical bonding forces spread over a long chain of amino acids. Success at making long polypeptides seemingly dooms the polymer to remain fixed to the surface instead of being released into solution as needed by life.
A major flaw with the studies described above is that they did not attempt to account for how homochiral selectivity could be enforced by this route. Synthesizing polypeptides with a racemic mixture of amino acids offers prebiotic life nothing in terms of functionality. For proteins to assume specific and reproducible structures, a homochiral set of amino acids must be used. With this goal in mind, one laboratory reported that enantiomeric pairs of aspartic acid preferentially adsorb to opposing faces with mirror symmetry of calcite (calcium carbonate). It was proposed that aspartic acid, having three functional groups (two carboxylic acids and one amine group), orients itself with homochiral selectivity to the exposed chemical groups of calcite. This follows logically to account for the ~90 percent chiral enrichment observed.
It works for aspartic acid in this case, but what about glutamic acid? It has the same functional groups but they are spaced apart a little differently. There is no guarantee that the corresponding chiral glutamic acid also binds selectively to the same surface. Sadly, this was not reported. Among the 20 amino acids, only 11 possess at least three functional groups required for appropriate spatial positioning to the mineral interface. The remaining nine have only two, one carboxyl and one amine group. This leaves little room for attaining homochirality for all amino acids as needed to construct proteins.
To give this laboratory credit, they did mention that alanine, valine, and lysine do not exhibit chiral selection on calcite. The lack of data for most remaining amino acids leads one to believe minerals in general are another dead end for the homochirality problem that OOL protein researchers are trying to solve. In contrast to the conclusions I draw from these observations, the authors were not dissuaded from making bold claims about how this model might help account for homochiral polymerization of amino acids on mineral surfaces in general.
Mineral Models to Select Ribose for RNA
The carbohydrate ribose, required to make RNA, presents a highly problematic situation. Ribose has four chiral centers, unlike amino acids with just one. Therefore, D-ribose found in RNA has seven other chemical partners differing in their spatial arrangement of atoms. Ribose has one advantage to assist the OOL researchers. It most often exists as six- or five-membered ring structures where the functional hydroxyl groups are positioned geometrically to one side of this chemical framework. Minerals such as rutile can accommodate hydrogen bonding, presenting an opportunity to preferentially bind ribose over its other carbohydrate competitors. Such a model has been proposed with the caveat that a third dimensional force must be applied to effect separation of ribose from the other sugars.
The first two dimensions are accounted for by quasi-planar interactions of sugar rings to the mineral interface. In simpler terms, this mineral needs to be applied to a separation technique called chromatography. The tighter binding of ribose, by virtue of its hydroxyl groups all pointing to the mineral surface, would permit stronger retention where the other sugars would proceed more rapidly through this chromatographic separation.
There are several problems with this innovative proposal. Setting up a chromatographic separation does not happen naturally. It requires a specific design where all molecules must enter the chromatographic medium simultaneously. In other words, an external agent is required to carry out this exercise. Assuming that chromatography proceeds as hoped, the last 5-carbon sugar eluting from the chromatographic medium will be D- and L-ribose. There is still a racemic mixture of ribose that must be contended with. Finally, as discussed in my earlier article, appropriately linking a nucleobase and finally phosphate to D-ribose is an extremely difficult task without the use of specifically engineered catalysts, e.g., enzymes. This is highly unlikely to happen in a prebiotic scenario.
The Task at Hand
I have addressed here the potential that minerals offered OOL researchers to abiotically synthesize the first functional biopolymers. Simple principles discounting these proposals can be applied to other comparable scenarios in this area, but that would require a much longer and more technical article. Suffice it to say there are sound counterarguments to the plethora of schemes that OOL researchers devise in trying to account for how life could have emerged abiotically. Critically examining these schemes is the job with which scientists on the other side of the field are tasked.