Intelligent Design
Life Sciences
“Remarkably,” Odor Code Just Doubled
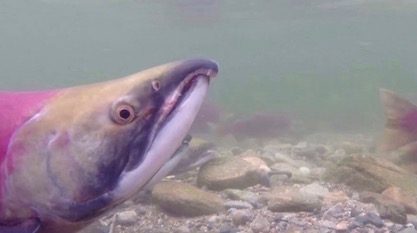
Though a distinct odor can instantly recall experiences of decades earlier, the sense of smell somehow doesn’t get the same respect as sight and hearing. We joke about political and other claims not passing “the smell test,” suggesting that something foul is afoot. But the mechanisms of olfaction, so welcome at holiday feasts and in a range of other contexts, are no less amazing than retinas and cochleas — and may be even more strongly suggestive of design. They rely on combinatorial codes generated by hundreds of inputs which are processed downstream to identify thousands of different odor molecules. Now, scientists have almost doubled the “alphabet” of input signals available to the processor.
Before proceeding, it might be worthwhile to review Illustra Media’s animation of olfaction from the film Living Waters:
Olfaction begins with molecular machines called Olfactory Receptors (ORs) embedded in the tips of cilia exposed to the environment. Fruit flies have 50 types of ORs coded by their genes; we humans have 400. The cilia are membrane extensions of Olfactory Sensory Neurons (OSNs) that will convey the information from the ORs to a processing center called a Glomerulus. There may be dozens or hundreds of glomeruli, depending on the species (humans have 1,200; dogs have a hundred times more). The specific wiring of OSNs to glomeruli, i.e., the attachment points (top, bottom, left, right, etc.) provides data for the combinatorial code. This is how a finite number of ORs can inform the human brain about a trillion odors, much like a finite number of alphabet letters can generate a vast number of words.
Up till now, research on olfaction assumed that the ORs acted in a binary fashion, like bits (on/off, 0 or 1). An individual OR either responded to its matching odor molecule, or it did not. This can be likened to yes/no questions about alphabet letters: Is the letter “A” present? (yes, no). The glomerulus would detect these binary inputs, depending on the corresponding OSN’s attachment point. The coding turns out to be richer than expected, as if the receptors can differentiate between upper and lower case letters. How this works is explained in an open-access paper in Nature Communications by Chinese and American scientists: “Odor-evoked inhibition of olfactory sensory neurons drives olfactory perception in Drosophila.”
It’s quite remarkable that scientists can monitor the firing rates of neurons at the pA range (one trillionth of an amp). The team measured spike patterns of OSNs in fruit flies — another remarkable achievement when you think how small those little bugs are. But they reserved the word remarkable for what they found:
Inhibitory response occurs throughout the nervous system, including the peripheral olfactory system. While odor-evoked excitation in peripheral olfactory cells is known to encode odor information, the molecular mechanism and functional roles of odor-evoked inhibition remain largely unknown. Here, we examined Drosophila olfactory sensory neurons and found that inhibitory odors triggered outward receptor currents by reducing the constitutive activities of odorant receptors, inhibiting the basal spike firing in olfactory sensory neurons. Remarkably, this odor-evoked inhibition of olfactory sensory neurons elicited by itself a full range of olfactory behaviors from attraction to avoidance, as did odor-evoked olfactory sensory neuron excitation. These results indicated that peripheral inhibition is comparable to excitation in encoding sensory signals rather than merely regulating excitation. Furthermore, we demonstrated that a bidirectional code with both odor-evoked inhibition and excitation in single olfactory sensory neurons increases the odor-coding capacity, providing a means of efficient sensory encoding. [Emphasis added.]
Here’s a new analogy to explain what’s going on. We shouldn’t think of OSNs as on/off switches, indicating the presence or absence of a molecule, but as triggers of two states, like 1 or -1. Think of a radio carrier frequency that can be modulated by a waveform going up or down. Olfactory receptors can apparently fire spontaneously, even when an odorant is not present. The rate of spontaneous firing provides the carrier frequency. When an odorant comes in, the OR can create an “excitation” signal or an “inhibition” signal to the downstream processor. What makes it signal one state or the other is not yet clear, but the OR triggers an inflow of calcium ions for excitation, or quenches the flow for inhibition. This effectively doubles the number of “bits” available to the olfactory code — i.e., its informational entropy.
As shown in Fig. 7b, the inclusion of odor-evoked inhibition in OSNs increases the information entropy of most OSNs and the total entropy of the whole system for all odor number tested (Fig. 7b, inset). Mechanistically, the inclusion of OSN inhibition increases the coding capacity of OSNs by preventing response saturation (Supplementary Fig. 7) and making the responses more uniformly distributed within the response dynamic range (Supplementary Fig. 8). The correlations in the OSN responses were studied by the PCA. A given principle component (PC) can be used for coding if its variance determined by its eigenvalue is larger than a detection (coding) threshold set by the noise level in the system. The number of PCs whose eigenvalue is above the coding threshold (set to be 1 in this study) defines an effective (independent) dimension for coding. As shown in Fig. 7c, the inclusion of OSN inhibition decorrelates odor-evoked responses across OSNs, thus increasing the independent dimensions of odor coding. Therefore, an OSN with both odor-evoked inhibitory and excitatory responses can compute and amplify the difference between similar odor mixtures as illustrated in Fig. 7a.
This puts more finesse into the code. The authors consider this a more efficient mechanism than previously thought, because it offloads some of the processing from the glomerulus onto the OSN.
A series of clever experiments led to their conclusions. They were able to breed flies with just one OR, and study what happened when controlled odorants impinged on the receptors. First, they found that inhibition could lead to the same behaviors as excitation. Second, they observed that one OSN could drive two opposing behaviors “and discriminate odor mixtures if inhibition and activation coexist.” When inhibition was suppressed, thirdly, the behavior (attraction or avoidance) switched.
Fourth, odor-evoked inhibition of OSNs increases odor-coding capacity by reducing response saturation and decorrelating odor representation. These findings establish that odor-evoked inhibition of OSNs is a primary odor code and that a bidirectional code with both inhibition and activation in the same OSN is an efficient coding strategy.
How can the same odor trigger two responses, excitation or inhibition? The authors suggested some situations that might determine the outcome. Since an OSN can have multiple ORs on its cilia, the particular ratio of OR responses might reach a threshold that switches the OSN between excitation and inhibition. It must be remembered, too, that odors often come in mixtures. Odor bouquets may contain 60 or more different molecules. Perhaps some odors or odor mixtures block transduction channels (inhibition) instead of exciting them. Odors might compete with each other for receptor attachment. Maybe ORs are sophisticated enough to switch between responses depending on circumstances. Whatever the mechanism, these findings show that the odor code is richer than thought.
Although they experimented with fruit flies, they believe this is a general principle for all animals. “This olfactory architecture is conserved from insects to mammals, suggesting a common solution to olfaction.”
Codes, information, efficient strategies — this is intelligent design territory. No wonder the team said nothing about evolution.
Image source: Living Waters, via Illustra Media.