Intelligent Design
Protein Folding and the Four Horsemen of the Axocalypse
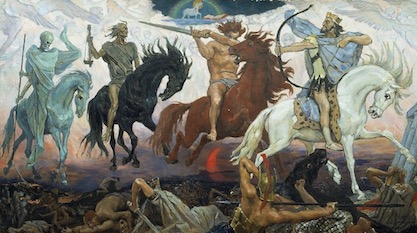
One of the most significant projects for the intelligent design movement was Douglas Axe’s research testing the rarity of protein folds. Axe’s method represented the most accurate approach for addressing the problem to date, but his was actually one in a line of studies which concluded that amino acid sequences forming the stable proteins found in nature are exceedingly uncommon. As a consequence, most proteins seen in life could never have originated via random mutations and selection.
One of the early online critics of Axe’s work was Arthur Hunt, who wrote a lengthy critique at the Darwinian advocacy site Panda’s Thumb. His area of expertise is not the evolution of new protein folds, and his arguments revealed a general misunderstanding of Axe’s approach and the field in general. The reviewers of Axe’s JMB article which reported his results were experts in protein studies, so they would have identified any of the issues raised by Hunt if they were real. Instead, they published the article affirming both the design of Axe’s experiment and his conclusions. A more recent series of critiques, published by the online journal Sapientia, was aimed at Axe’s book, Undeniable: How Biology Confirms Our Intuition That Life Is Designed. The four biologists offering these critiques I affectionately refer to as the four horsemen: Dennis Venema, Cara Wall-Scheffler, Joel Duff, and Keith Fox. As Axe pointed out in his response to these critiques, they mostly ignore the main argument of the book — a common-sense argument — choosing to get lost in scientific details instead. Here at Evolution News, Axe has responded specifically to Venema’s critique of his protein work.
Here, I want to flesh out some of the salient details of this discussion.
Faith in Natural Selection
The primary content of these four critiques was a reaffirmation of the critics’ faith in the unlimited power of natural selection to generate all of the complexity seen in life. A secondary argument was that people’s design intuition could be fooled by such artifacts as the Corona Arch which is a rock formation in Utah formed by erosion over extended periods of time. This common response represents a fundamental misunderstanding of the argument for design which has been corrected many times in the past. At its foundation, it fails to recognize that objects of intelligent design typically demonstrate a highly improbable configuration of very different units which transcends their physical and chemical properties.
For instance, the arrangement of parts in a car is not primarily associated with the chemistry of rubber or the physics of metal. Instead, it reflects the purpose for which the car was intended. In the same way, the global arrangements of tissues and their constituent molecules in a camera-like or compound eye are not entirely explainable by chemistry and physics. Some aspects of tissue behavior can be the product of biomechanical (physical) forces, but the overall structure of the eye can only be explained by the engineering constraints that allow for the purposeful goal of vision. Likewise, the sequential arrangement of amino acids that results in the proper folding of a complex protein cannot solely be explained by the chemistry of the building blocks, but it can only be fully understood by the function which the protein fulfills, such as driving forward some key chemical reaction or forming a structural element in a molecular machine.
Another criticism championed by Venema argues that proteins are not as rare as Axe’s research implied. Others have made similar claims by citing experiments that explored random libraries of amino acid sequences to identify the portion that demonstrated some “function.” For instance, one referenced study showed that roughly one in a trillion chains could bind (stick) to ATP, and some were identified that could catalyze (accelerate) ATP’s breakdown to ADP. Likewise, Venema referenced research on random sequences inserted into the genome of a bacterium and on an orphan gene discovered in yeast. At first glance, these purported counterexamples appear to challenge Axe’s results. However, a careful study of the physics and chemistry behind protein folds quickly reveals that the critiques’ true nature is little more than smoke and mirrors.
Of Smoke and Mirrors
To appreciate their fundamental flaws in reasoning, one needs to understand the science behind protein folding in general and enzymes in particular, since actual enzymes were the focus of Axe’s and previous studies. A protein’s amino acid sequence contains the information for both its folded three-dimensional structure and the folding pathway for the initial chain to form into the final shape. Folding often begins spontaneously with different sections of the chain forming into three-dimensional shapes, most commonly alpha helices and beta sheets. These secondary structural components then fold into the tertiary structure which tends to come in chunks known as protein domains. Multiple domains can then combine into the final configuration which contains an active site that catalyzes one or more chemical reactions.
The active site is composed of amino acids from distant locations on the unfolded chain brought into close proximity through the folding process, so their side chains can serve several key functions. Some create a chemical environment that differs dramatically from that of the surrounding water, and the manufactured local conditions allow for reactions to proceed that would otherwise have been impossible. Other side chains form into sites that bind to target molecules and position them at the right locations and in the proper orientations in the active site. Still others drive specific chemical reactions. In summary, the active site functions as an atomic-scale factory which reengineers molecules through performing high-precision nano manipulations.
I recently stumbled upon a video of the enzyme aconitase which animates this process in stunning detail:
The enzyme performs several tasks:
- It binds to citrate, placing this substrate molecule at a precise location in a precise orientation. A neighboring iron-sulfur cluster helps stabilize the substrate electrostatically.
- Histidine 101 donates a proton to remove one OH group from the citrate.
- Serine 642 acts as a base by accepting a hydrogen from another location in the substrate.
- The interactions between the enzyme and the altered substrate flip the substrate upside down.
- Histidine 101 binds to a proton from a passing water molecule, causing OH to attach to a new location in the substrate.
- Serine 642 returns a hydrogen atom to a new location in the substrate. The enzyme returns to its original atomic structure.
- The enzyme releases the substrate, so the enzyme can act on another citrate molecule.
Equally dramatic, many enzymes couple multiple reactions such as the energetically downhill breakdown of ATP into ADP with a second reaction that would otherwise be energetically unfavorable. This coupling is essential to life since the energy from the downhill reaction must be used to drive the other reaction uphill to overcome its free-energy barrier. A prime example is the group of enzymes known as aminoacyl-tRNA synthetases (aaRS) which enable the translation of RNA (to make proteins) by performing the following steps:
- The enzyme’s active site binds to an amino acid and an ATP molecule.
- The aaRS breaks ATP into AMP and pyrophosphate (PPi) and chemically bonds the AMP to the amino acid. The PPi leaves the enzyme.
- The aaRS binds to a tRNA and then bonds the tRNA to the amino acid while breaking off the attached AMP.
- Finally, the amino-acid-tRNA complex (aminoacyl-tRNA) is released, and the enzyme then returns to its original state.
A high-energy phosphate bond in ATP was used to allow for the linking of the amino acid to a tRNA which would otherwise have been energetically unfavorable.
These examples demonstrate how enzymes commonly perform exquisitely orchestrated targeted reactions which require highly specific amino acid sequences. In addition, they highlight the irrelevance of research cited by critics to challenge Axe’s conclusions. For the actions performed by randomly generated or short amino acid chains are qualitatively different from the “mechanistically enzyme-like” activity at such complex active sites.
Rubber Slingshot or High-Performance Rifle?
As an analogy, imagine you are asked how long a child would take to create a functional weapon out of scrap pieces in a junkyard. The answer depends on the precise definition used for “functional weapon.” If one means anything useful for defending oneself, the answer would be not very long. A large piece of rubber could be used as a slingshot against an annoying little brother at close range. However, if one meant a fully operational high-performance rifle with a laser-guided scope, the answer would be never. The functions identified in the random-sequence experiments or in short chains are relatively generic, much like a crude slingshot. In contrast, a complex enzyme such as aconitase is more like a high-performance rifle.
In addition, the active sites in complex enzymes, particularly those which perform multi-step reactions, cannot slowly evolve from random sequences as claimed in Fox’s essay. Their chemical activity only commences after specific side chains from key amino acids scattered throughout the protein are brought together to their proper final locations with atomic precision. This accuracy requires that substantial information be encoded in the protein sequence from the beginning.
Deniers of protein rarity often attempt to argue against the need for such exacting constraints in enzymes by citing the discovery of “intrinsically disordered” proteins which do not immediately lock into well-defined shapes. Critics claim such examples demonstrate that proteins do not require highly specific folding to initiate their targeted activities. The problem is that these proteins do not perform the same functions as typical enzymes, so they operate according to different constraints. They actually do fold in order to function, but they have the optimal amount of “looseness” to perform their intended tasks. Consequently, they are in fact highly designed. In a paper that deals with these “intrinsically disordered proteins,” the authors note that the levels of these proteins within cells are “tightly regulated to ensure precise signaling in time and space, and mutations in [them] or changes in their cellular abundance are associated with disease.” In other words, their sequences are highly specified for their particular functions, and mutations to them cause disease.
As a specific example, Venema referenced the BSC4 gene which is imagined to have originated relatively recently through undirected mutations in non-coding DNA sequences. Others have pointed out that the BSC4 protein forms into a “partially specific three-dimensional structure,” and they imagine that it could eventually evolve into a more rigid native fold. The problem is that both the undirected nature of the gene’s origination and its possible future as more tightly folded were not proved but only assumed. The whole point of the discussion is the question of whether new proteins were generated by undirected processes, so one cannot presume that claim at the beginning. And BSC4 appears fully functional, so it is likely already highly optimized. The challenge is for materialist scientists to separate what might be true in their imaginations from what has been demonstrated empirically.
An Additional Common Error
An additional common error by critics is to cite experiments that either enhance an existing weak function in an enzyme or produce some trivial new activity such as binding. Such modest changes never result from a new protein fold, but they instead represent minimal adjustments to an existing one. In stark contrast to the claims of critics, experts have stated that evolving truly novel folds is exceedingly difficult and beyond modern science’s current understanding.
Thus, Doug Axe’s work illustrates the rarity of protein folds capable of carrying out enzyme functions. If the four horsemen want to critique the work on scientific grounds, they need to compare apples to apples, enzymes to enzymes, and demonstrate, rather than assume, that such complex functions can evolve. If they want to claim that “intrinsically disordered” proteins have no structure and thus demonstrate that Axe’s measure is an overestimate, they should perhaps measure how difficult it is to get such an intrinsically disordered protein from random sequences. If they want to disagree with the common science approach, then perhaps they should set aside the pre-conceived notions of evolutionary biology and try some common science for themselves.
Image: Four Horsemen of Apocalypse (1887), by Viktor Vasnetsov [Public domain], via Wikimedia Commons.