Evolution
Intelligent Design
Design in the First Animals
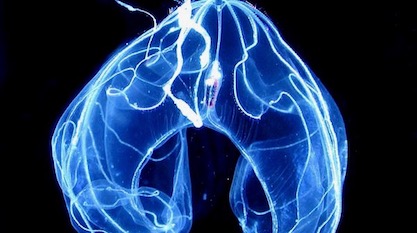
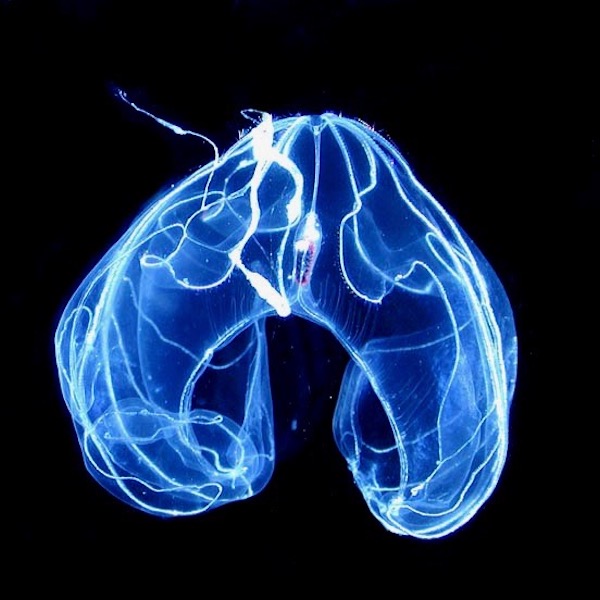
It didn’t take long for animals to master physics and engineering. The first animal body plans were performing feats that fascinate — and baffle — research scientists.
Ctenophores: Flashing Paddles
Popularly called comb jellies, ctenophores (pronounced TEN-o-fours) are usually small, centimeter-sized marine organisms, although some species are close to a foot long. They are furnished with rows of cilia, called comb rows or ctenes, which function as paddles for swimming. Though gelatinous and transparent, comb jellies are unrelated to jellyfish (phylum Cnidaria); they have been classified into their own phylum, Ctenophora, characterized by eight of these comb rows. Scientists debate whether ctenophores are the earliest animals that appeared in the Cambrian explosion, as opposed to sponges (phylum Porifera). If so, they arrived with multiple tissues, a nervous system, and a digestive system. That’s a lot to account for without any known transitional forms.
Up close, comb jellies look like alien spaceships with flashing lights. Rainbow colors race down the comb rows as the cilia beat in series. There’s a puzzle: how do the cilia coordinate their movements? A recent paper in Current Biology by Jokura et al. shows it’s not simple.
Japanese scientists from the University of Tsukuba identified a structural protein, CTENO64, that is required to orient each cilium within the “compartmenting lamellae” that hold the comb rows together. Without the protein, the animals couldn’t swim. This protein, unique to ctenophores, is “unlike any protein studied to date,” they say. To add to Darwin’s stomach pains, the ctenophores found in the Cambrian fossil record look just like the ones found swimming today, implying that this protein and the unique comb row structure it supports appeared with a bang and then didn’t evolve for 520 million years. Some fossil comb jellies, in fact, were even more complex than modern ones, possessing armored shields.
In a review article in the same issue of Current Biology, Sarah P. Leys compares the structure of comb rows to feathers.
Consider the feather. It’s light yet strong and incredibly effective as a paddle. Yet unlike man-made paddles, it is made of hundreds of thousands of filaments. In fact, most biological structures that function as paddles, moving either air or water, are made of highly aligned filaments. Cilia are essentially tiny filaments that project from the surface of cells. They arise singly or in groups from a single cell and when in groups, the distance between the cilia is what makes them function as a paddle or a rake. [Emphasis added.]
The spacing and orientation of each paddle must be regulated, she goes on to say. Picture rowers in a boat paddling in random directions out of sync; they’d get nowhere fast. “If any animal should have sorted out how to organize cilia properly it’s the ctenophore,” Leys comments. Indeed, the ctenophore did sort it out (or rather, was given a well-sorted structure at the beginning). The compartmenting lamellae are just the right shape to organize the cilia into well-ordered arrays. CTENO64 orients each cilium properly so that they can beat in sync by “hydrodynamic coupling,” something like crowds doing the Wave in a stadium.
But even that’s not the whole design story. As Michael Behe showed in Darwin’s Black Box when he introduced cilia as irreducibly complex biological machines, and elaborated in The Edge of Evolution, each cilium functions because of the precise arrangement of molecular motors and tracks inside the filament. Leys describes the hierarchical arrangement:
Cilia themselves are typically well-organized structures: each cilium consists of a ring of nine microtubule pairs surrounding a central pair (together called the axoneme), all enclosed by the cell’s membrane. In the ctenophore, thousands of cilia are arranged hexagonally in a structure called the comb plate, and the entire comb plate beats as one.
A micrograph shows the cilia arranged in order like a honeycomb, each one with its ten microtubule pairs. From molecular motors to cilia to lamellae to complete rainbow-flashing organism, it’s design all the way up.
Mollusks: Structural Strength and Beauty
You’ll love the short video here from the University of Michigan:
Assistant Professor Robert Hovden practically glows with inspiration about nacre, or mother-of-pearl, produced by “simple” mollusks. Nacre is nature’s toughest material: lightweight but nearly impossible to crack with the hands, even though it’s composed of the same material as chalk. Nacre’s combination of hardness and resilience has mystified scientists for eighty years — until now.
“We humans can make tougher materials using unnatural environments, for example extreme heat and pressure. But we can’t replicate the kind of nano-engineering that mollusks have achieved. Combining the two approaches could lead to a spectacular new generation of materials, and this paper is a step in that direction,” said Robert Hovden, U-M assistant professor of materials science and engineering.
Researchers have known the basics of nacre’s secret for decades — it’s made of microscopic “bricks” of a mineral called aragonite, laced together with a “mortar” made of organic material. This bricks-and-mortar arrangement clearly lends strength, but nacre is far stronger than its materials suggest.
By studying electron microscope images of nacre flexing in real time, Hovden’s team observed proteins stacked like the bricks of a building, called “tablets,” which are bonded with thin protein mortar between courses. They watched what happened when strain was applied and released.
They found that the “bricks” are actually multisided tablets only a few hundred nanometers in size. Ordinarily, these tablets remain separate, arranged in layers and cushioned by a thin layer of organic “mortar.” But when stress is applied to the shells, the “mortar” squishes aside and the tablets lock together, forming what is essentially a solid surface. When the force is removed, the structure springs back, without losing any strength or resilience.
Some man-made materials like plastic can recover their shape, but not completely. They lose strength with each iteration. “Nacre lost none of its resilience in repeated impacts at up to 80 percent of its yield strength,” they found. All this in a creature that dates back to the Cambrian explosion!
“It’s incredible that a mollusk, which is not the most intelligent creature, is fabricating so many structures across so many scales,” Hovden said. “It’s fabricating individual molecules of calcium carbonate, arranging them into nano-layered sheets that are glued together with organic material, all the way up to the structure of the shell, which combines nacre with several other materials.”
The structure of some mollusk shells, like the chambered nautilus, arouse awe with their exquisite beauty and mathematical precision. Hovden’s engaging story is only spoiled momentarily by a bit of Darwinian nonsense tacked on as if by a sense of obligation:
“Nature is handing us these highly optimized structures with millions of years of evolution behind them,” he said. “We could never run enough computer simulations to come up with these — they’re just there for us to discover.”
Crustaceans: Memory Smarts
A maze is for mice, most people think. Who would put a crab in a maze? Scientists at Swansea University did that, New Scientist reports; and what they found surprised them.
A species of crab can learn to navigate a maze and still remember it up to two weeks later. The discovery demonstrates that crustaceans, which include crabs, lobsters and shrimp, have the cognitive capacity for complex learning, even though they have much smaller brains than many other animals.
“Crustaceans have a brain roughly 10 times less than the size of a bee’s in terms of neuronal count,” says Edward Pope at Swansea University in the UK.
In mazes involving five changes of direction, the test crabs took a few weeks to learn how to find the reward. Once they learned the route, they remembered it after a two-week gap, even without a treat at the end.
Some of the Cambrian animals resemble crabs. It’s not possible to determine whether the first arthropods and crustaceans possessed problem-solving intelligence and memory, but it’s a fair inference to believe that they could, Pope implies:
“This study is important because we know that insects, especially ants and bees, have some impressive mental abilities but we haven’t really looked for them in their aquatic counterparts. The fact that crabs show a similar ability to insects is, in some ways, not that surprising but it is great to be able to show it so clearly.”
Cnidaria: Jellyfish Superpowers
The oldest jellyfish fossil found so far dates to 500 million years ago, but since the record may be biased against soft-bodied organisms, older ones could conceivably be discovered. Some cnidarians (the phylum including corals, jellyfish, and sea anemones) do go back farther into the Precambrian. The earliest jellyfish was clearly a jellyfish. Like most other Cambrian fossils, they exploded onto the scene, with complex stinging cells and all.
Jellyfish have “superpowers” according to news from Tohoku University: notably, the ability to regenerate body parts. Have humans “devolved” that ability?
Cnidarian jellyfish — named for their stinging cells — have existed on the earth for more than 500 million years. They form part of a unique group of animals that are not bilaterally symmetrical and also possess the capacity to regenerate body parts — a trait most of the complex animals, including humans, have lost.
Regeneration is clearly more complex than speeding up cell division. Cells need to know where to go. They must follow a blueprint to grow and differentiate into the tissues and organs that need replacing.
To investigate the role of cell proliferation following food uptake in determining body-size growth, appendage shape, and regeneration in Cladonema jellyfish, the researchers examined the distribution of cells that play a key role in DNA replication through cell division, producing new ‘daughter’ cells that are identical to the original ‘parent’ cell. They found spatially distinct groups of proliferating cells in the medusa (sexual) life-stage, with cell proliferation in the umbrella-shaped portion of their body being uniform, while cell proliferation in the tentacles was clustered.
After withholding food or blocking cell proliferation using a cell-cycle inhibiting agent, the researchers found body size growth was inhibited, and they also observed defects in tentacle branching, differentiation of stem cells into stinging cells, and regeneration. These results suggest that free-swimming adult jellyfish in the sexual stage possess actively proliferating cells that play a key role in controlling body-size, tentacle shape, and regeneration.
Foresight and Planning
So, from from both fossil and living organisms, it’s clear that complex organs and behaviors seen today in arthropods, crustaceans, mollusks, and comb jellies have roots in the first appearances of these animal groups. Some diversification has occurred subsequently, and even some devolution. Working systems from the beginning, however, are best explained by foresight and planning.
Photo: Bathocyroe fosteri, a ctenophore, by Marsh Youngbluth [Public domain], via Wikimedia Commons.