Intelligent Design
Physics, Earth & Space
Do We Live on a Privileged Planet?
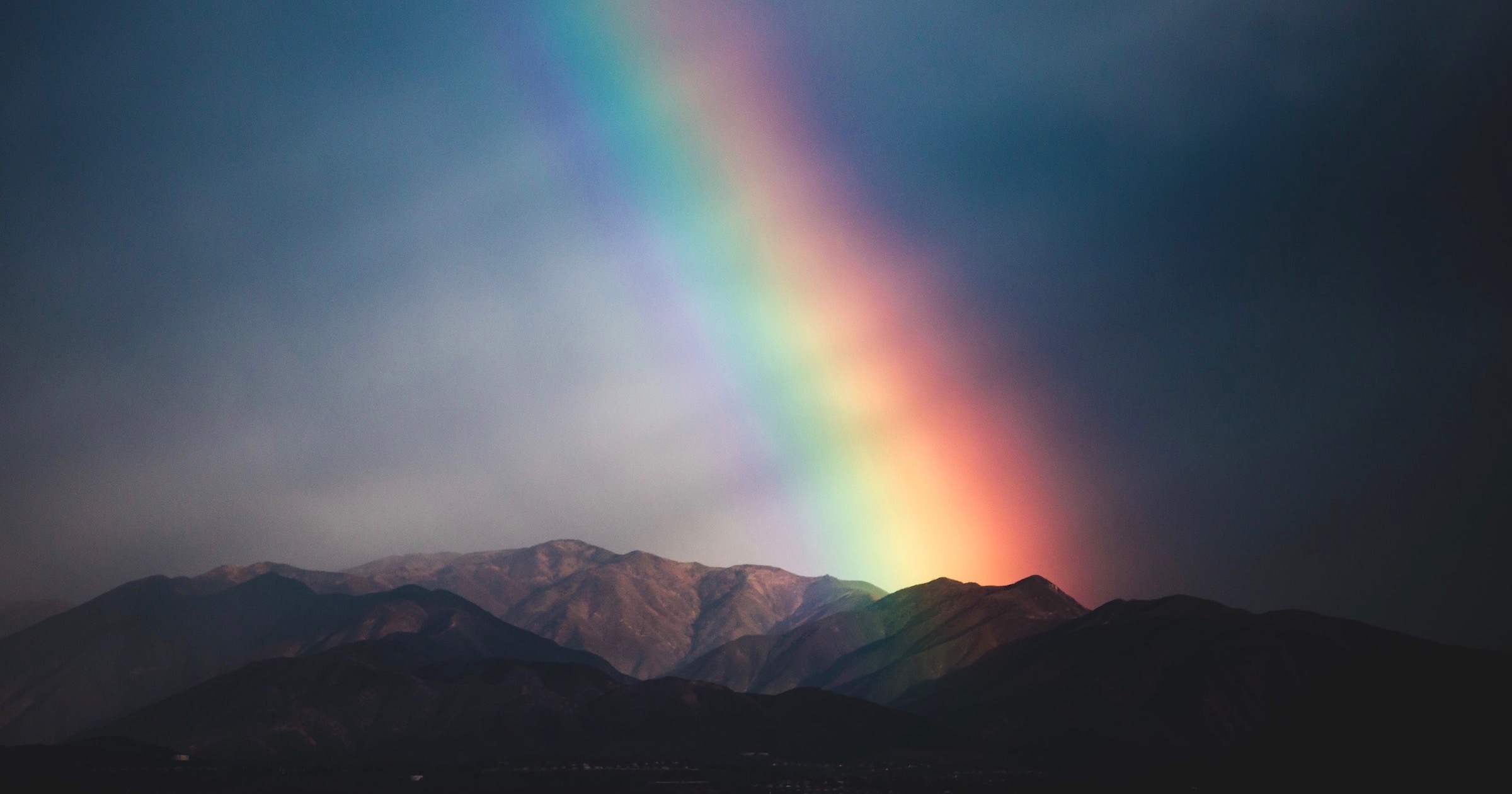
Editor’s note: This excerpt is from a chapter in the newly released book The Comprehensive Guide to Science and Faith: Exploring the Ultimate Questions About Life and the Cosmos.
The gratuitous beauty of the starry heavens above. The chimerical appearance of rainbows. The austere splendor of solar eclipses. These inspire people in every time and place. For most of human history, they were also mysteries.
In a sense, we’ve now removed many of the mysteries. Scientists routinely measure the distances to the stars. We know how sunlight passing through millions of suspended water droplets in the atmosphere produces a rainbow. We can predict the timing and location of solar eclipses years in advance anywhere on Earth to within one second.
Yet these discoveries point to even deeper mysteries. Why is our world, from our local and galactic environment to the constants of physics, set up so that we can see the stars, rainbows, and solar eclipses? After all, our ability to see these things is not logically required for our existence. Surely, the universe could have been otherwise.
A Cosmic Conspiracy
This is the question I set out to answer with my coauthor, Jay W. Richards, in our book The Privileged Planet: How Our Place in the Cosmos Is Designed for Discovery. The subtitle gives away our argument. We argued not just that the universe is vaguely habitable and open to science, but that those rare places in the universe best suited for complex life and observers are also the best places, overall, for scientific discovery (hereafter, the PP thesis). Moreover, we argued that this is evidence of a cosmic conspiracy rather than a mere coincidence. We set out to test this hypothesis against the best evidence from natural science. Together, these discoveries — that is, observations — form a cumulative case in favor of our hypothesis. We didn’t try to immunize our argument against future discoveries, but rather, sought to put it at risk. That’s why we predicted that relevant new observations (or new analyses of existing observations) would confirm our argument.
In the present book, the chapter “How Do Solar Eclipses Point to Intelligent Design?” describes a small bit of the evidence for the PP thesis. The topic of total solar eclipses was the first instance of the PP thesis that I discovered, and it is the topic of the first chapter in The Privileged Planet. Since the PP thesis is a cumulative argument, it becomes stronger with each instance that is added. Given the limited space available, I will discuss only two additional instances in this chapter: the visibilities of rainbows and the stars.
Rainbows and Eclipses
In The Privileged Planet, I ended the chapter on solar eclipses with a teaser on rainbows. How are rainbows like total solar eclipses? They’re both beautiful visual spectacles (eye candy!) requiring no special optical aids to enjoy. We don’t need to be able to see either one for our survival. Rainbows are far more common than total solar eclipses, but rainbows are rare in regions of the world with infrequent rainfall. From my experience, I see several rainbows a year on average; whenever I’m with others when I do see one, I hear that they never tire of the sight. Both rainbows and total solar eclipses are fleeting phenomena, typically lasting minutes.
For a rainbow to appear, you need suspended water droplets in the atmosphere and the direct sunlight that results from the sun being between the horizon and 42 degrees altitude. This typically occurs just after a thunderstorm has passed and small droplets are still in the atmosphere, and the sky is clearing in front of the sun. Seems like a simple setup. This must be a common phenomenon in the cosmos, right?
Let’s consider the other major bodies in the solar system — the planets and large moons. Mercury, the closest planet to the sun, and the moon look superficially similar. This is because they lack atmospheres. Can’t have rainbows there. Same goes for Mars. Even though it has a thin atmosphere, it’s too dry. It never rains. On the other hand, Venus has too much atmosphere. It is perpetually cloud-covered, and its surface is bone-dry. The giant planets aren’t options because they have no solid surfaces. What about their moons?
The only moon in the solar system with a substantial atmosphere is Titan. Its atmosphere is dominated by haze. From the ground, the sun would be faint and the sky orange and hazy. Hypothetical Titans would not see rainbows either.
Life requires liquid water. Liquid water in the form of precipitation is required to make rainbows. A rich biosphere powered by photosynthesis needs not only abundant water, but also abundant sunlight. Precipitation is important, too, in order to permit life to flourish on the continental interiors. The link between rainbows and life is obvious.
What Good Is a Rainbow?
Yes, rainbows are beautiful, but are they good for anything? Indeed, they have been very important for science, but in subtle ways. Rainbows have long intrigued casual and careful observers alike, and they’ve prompted many questions. How are they produced? Are the colors of the rainbow already present in the sunlight? Is it possible to make an artificial rainbow in the lab? Over the centuries, these and other questions have spurred research in optics and the physics of light.
Descartes and Newton performed early experiments with prisms to produce artificial rainbows. These were the first spectroscopes — instruments that spread light into its constituent colors, its spectrum. These could be made to spread out the spectrum of any light source over a larger range of angles than a natural rainbow, permitting more detailed examination. Indeed, when astronomers pointed their spectroscopes at the sun, important new discoveries were made. In 1802, English chemist William Hyde Wollaston discovered a series of dark lines in the solar spectrum when viewed at high resolution. Joseph von Fraunhofer rediscovered them in 1814. We now know them as Fraunhofer lines.
The application of the spectroscope to the fields of astronomy and chemistry led to enormous leaps in our understanding of the universe. Spectroscopists learned that each chemical element has a unique spectrum, a kind of fingerprint. This powerful tool permitted astronomers to identify the chemical elements present in the sun’s atmosphere. In 1864, William Huggins joined a spectroscope to his telescope and obtained spectra of distant stars. Spectroscopists measured their compositions and other properties as well, such as temperature and density. Jules Janssen discovered the second most abundant element in the universe, helium, in the spectrum of the sun in 1868 during a total solar eclipse. Astronomers also learned that they could measure the velocity of a star along the line of sight using the Doppler effect.
It’s as if someone has been trying to get our attention with a pretty shiny object writ large across the sky, saying, “Look here, stupid! This is important!” In other words, the beauty of rainbows attracted our attention, and when smart people thought carefully about them, our knowledge of nature expanded greatly. A partly cloudy atmosphere with precipitation gives us rainbows and permits us to observe solar eclipses. Our kind of atmosphere also permits us to view the distant stars.