Intelligent Design
Life Sciences
The Hidden City
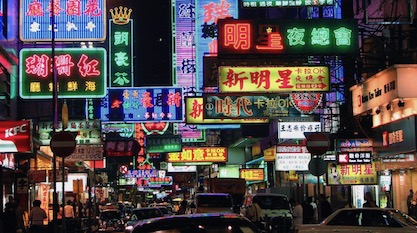
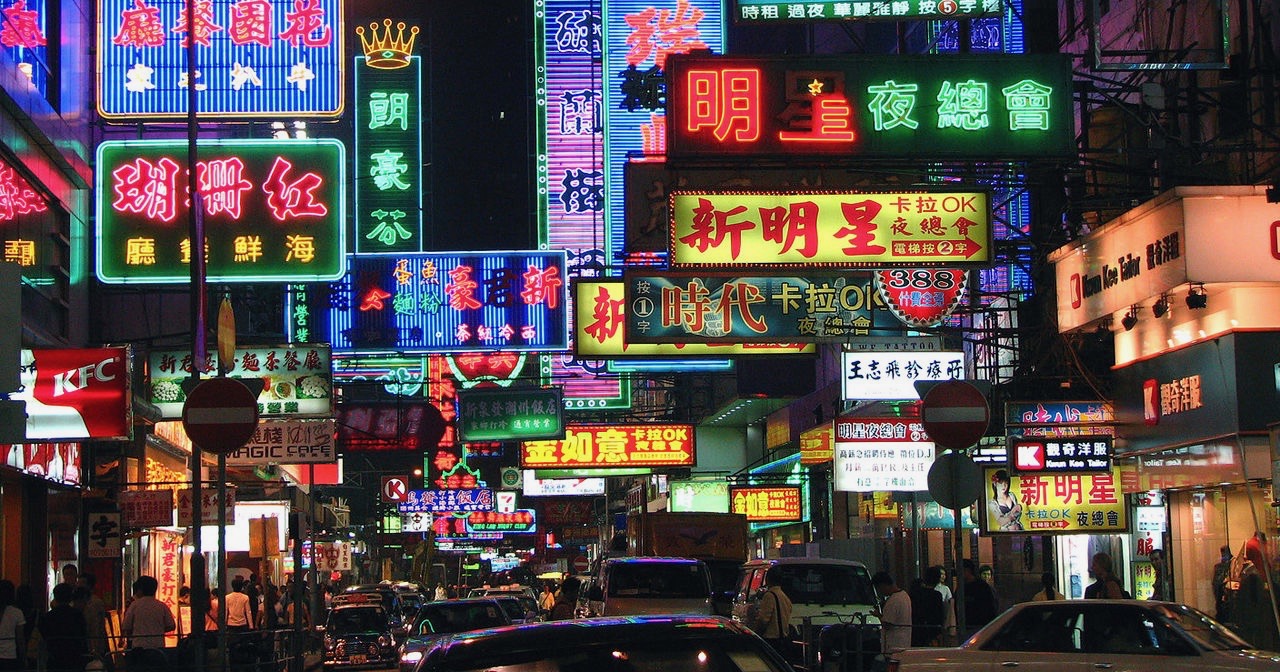
Picture a hidden city, that though it cannot be seen, is everywhere. Sound crazy? It’s real. And it is the most antic, madcap, crowded yet fantastically efficient city you could ever picture. It’s like Hong Kong sped up to an almost unimaginably manic pace, with all kinds of independent, apparently purposeful activities going on — fast, fast, fast! — conducted by a huge cast of actors (enzymes and other intricately sophisticated molecular machines made of proteins) that go about their business as if it were their business. There, I gave it away. This mysterious city I write about is a microscopic cell, made of DNA, RNA, proteins, and membrane. No doubt you were taught to think of a cell more or less statically, but it is a highly dynamic ever changing entity. How is all this activity coordinated and directed? The answer remains largely mysterious, and the more we find out the more the mystery grows.
Grand Central Library of the Cell
We do know this much. The nucleus is where DNA, the cell’s information storage system, resides. It serves as the cell’s Grand Central Library, where a good deal of the coordination takes place. DNA, the chief orchestrator, looks like a tangled mess, but it isn’t, it’s quite organized. It has to be. Supercoiled DNA packs tightly against the nuclear wall, inactive. Nearer the center, active chromosomes stake out territories, so that in the center, their unwound loops of DNA can partner with others in an intricate dance. Clouds of signal molecules surround these loops, looking for binding sites near genes. Most genes have multiple binding sites near them. When occupied, the binding sites send signals — yes, no, no, yes, yes — that get integrated into one overall signal. When it adds up to yes! a cascade of events begins — another kind of binding protein sits down on the DNA like a rider in a saddle, right in front of the gene, and attracts other proteins to itself, one by one. Then the cluster attracts a wandering machine called an RNA polymerase, which will copy (transcribe) the DNA into RNA. The whole complex waits like a race horse in the starting gate until the signal is given, then bang! the polymerase whizzes off, transcribing DNA into RNA at an astonishing clip of 30 nucleotides per second.
Sometimes the polymerase jumps between strands, forming an RNA made from two separate chromosomes. Sometimes polymerases racing in opposite directions run into each other, like Keystone cops. And sometimes polymerases run into what are called replication forks, the places where DNA is being duplicated in order for the cell to divide. The RNA polymerase politely steps aside.
You are probably wondering what the RNA is good for. It gets processed and shipped out to the cytoplasm, where it is turned into proteins like the polymerase and binding proteins, or the thousands of other proteins the cell requires. Proteins are the actors that accomplish things in the cell, and the building blocks from which things are made. You will meet other examples as we go.
A Conundrum
Here’s a conundrum, though. These proteins are all specified by the DNA — as we have seen, DNA is copied into RNA, then RNA is translated into protein. Consequently, proteins cannot exist without DNA. However, if you think about it, DNA cannot exist without proteins either. For example, to replicate DNA, one protein unwinds the DNA, creating a fork with two strands; another protein duplicates the strand to the right, while another casts off loops from the strand to the left so it can be copied backward (!); then another stitches the left-hand strand together. Meanwhile, thirty or so other proteins keep watch over the DNA, proof-reading, correcting, and ensuring very few errors — about 1 mistake per billion nucleotides copied. It’s a chicken and egg problem — which came first, proteins or DNA?
Another Puzzle Remains
Even if that problem could be solved, another puzzle would remain — how the link between DNA, RNA and protein came about. We know how it’s done — ribosomes — just not how it came to be. Born in the nucleus, ribosomes are indispensable, efficient, self-correcting, decoding machines and protein factories. They are made of proteins woven together with RNA into tangled knots that somehow work together. Out in the cytoplasm where they do their work, a messenger RNA finds a ribosome and feeds through it like ticker tape. The ribosome reads the message as it feeds through and translates it to amino acids by matching the message to the right transfer RNAs (tRNAs), sliding each tRNA into place — click, click, click — and then stitching together the amino acids they carry to make a protein. (That went by fast!) Though not as fast as transcription, the ribosome manages a respectable rate of six to nine amino acids per second.
There is much more to this story than I have time to tell — suffice it to say that the genetic code, its replication, transcription, and translation, are at the center of life: They are interdependent highly optimized processes that are essential to life.
But these processes are not the only highly optimized ones in the cell. Mitochondria are the microscopic power plants of the cell whose sole purpose is to take glucose and convert it to ATP, the cell’s energy currency. Resembling miniature blimps with corrugated double membranes, they carry out an interlocking series of chemical reactions that squeeze out every last possible ATP from the breakdown of glucose. It’s a highly efficient, environmentally friendly process. Everything is recycled — one part of the process is called the citric acid cycle because it regenerates itself with each new round. Even the last high-energy electrons from the breakdown process are not wasted: a chain of proteins in the inner membrane passes these electrons like little hot potatoes from one to another, causing the energy of each transfer to pump hydrogen ions across the membrane, so that a molecular machine called ATP synthase can take advantage of the hydrogen gradient to create even more ATP. Like a turbine in a hydroelectric plant, ATP synthase lets the hydrogen ions flow back across the membrane through itself, rotating as the ions pass through, and generating ATP.
Do the Math
Overall, if you do the math, for every molecule of glucose that is broken down, 30 to 32 ATP are produced, representing an efficiency of about 40 percent. That’s two to four times better than an automobile engine and about as good as the best of all our power-producing methods except hydroelectric power.
Now let’s zoom through the constantly bustling, very crowded byways of the cellular city to see how that energy is used. Microtubules span the cell in all directions like reinforcing beams, serving as structural supports and highways. Microfilaments, delicate webs of protein, lie under the cell’s membrane to give it shape. The formation of these structures requires energy (ATP). A maze-like network of membranous tubules (with the unwieldy name of endoplasmic reticulum) stretches out from the nucleus, some with ribosomes embedded in them. As the ribosomes manufacture proteins (using more ATP), they are extruded into the tubules. The tubules then bud off little vesicles to be transported (more ATP) to a large layer of stacked pancake-like membranes between the nucleus and the surface of the cell — this place, the cell’s post office (also called the Golgi apparatus) is where proteins get processed and sorted for delivery, using ATP.
Outside, the cytoplasm is packed with hundreds to thousands of mitochondria (the power plants we discussed before) and free-floating ribosomes (protein factories). Ramifying networks of signal molecules transmit information from the cell surface to the nucleus and back again. Enzymes (a kind of protein) manufacture and tear down structures and compounds the cell needs, literally reshaping the cell to order (using yet more ATP — I’m going to stop here, you get the idea). Messenger RNAs look for ribosomes, newly minted proteins are chaperoned through the process of folding correctly (you don’t want to get that wrong — the consequences can be severe: Alzheimer’s is a due to misfolded protein), and clean-up crews mop up incomplete proteins and degraded RNA. Short and long interfering RNAs pair with complementary RNAs — this attracts slicer and dicer patrols looking for RNAs foolish enough to be caught double-stranded, to chop them up into nucleotides. I could go on. But I will give you just one more — kinesin.
Delivery for You, Ma’am
Kinesin has an odd shape — two long legs, two feet, and a stalk-like body — that is eminently suited for its work. When it receives a package to carry, it attaches itself to one of the many microtubule highways of the cell and begins to stride, one foot over the other, dragging its cargo behind it and looking for all the world like a little stick man walking down a road with a great big bundle on his back. Kinesin is the UPS delivery man of the cell — a molecular motor that can muscle its way through the jostling tangle of the cytoplasm, dragging its cargo with it. Because of its amazing tenacity kinesin is rarely dislodged, placing one foot securely after the other; by working in teams it is able to transport cellular objects as large as mitochondria. When it encounters an obstacle it can’t get around, kinesin will first recruit more kinesin motors to help. Failing that, it partners with another motor traveling in the opposite direction. They rock back and forth taking turns pulling in opposite directions, like a driver caught in a snow drift, until finally they dislodge their cargo. Then the original kinesin resumes travel in its old direction.
How does kinesin know which package to carry and what road to take? Apparently there are labels that kinesin recognizes, saying “Carry me!” but no one knows what the map is or how kinesin reads it.
How You Grew
I’ve shown you how a cell is like a city — but there is one special thing your cells do that a city can’t. At some point in your history you were a single cell that grew and became two, then four, then eight, all the way to a hundred trillion cells. All those cells remained one — a single individual. To do this, your cells specialized, some in manufacture, some in transport, some in signaling, some in energy storage, and some in waste management. All those cells now live together as a stable, cooperating, whole organism. Here’s the amazing thing — they do it because it is their nature, for the good of the whole. Absolutely no selfishness is possible here or all perish — rogue cells that defy the rules and break free to live without restraint are known as cancer. So cells are madcap, frenetic, bustling, efficient places — but places with rules. The final rule is this: the good of the whole before my own good. Not a bad rule to live by.
Photo: Portland Street in Hong Kong, by UCLARodent at English Wikipedia [CC BY-SA 2.5], via Wikimedia Commons.
Cross-posted with permission from Dr. Gauger’s website, Making Notes of the Moment.