Evolution
Intelligent Design
Studies on Insect Wings Validate Engineering Models for Adaptation
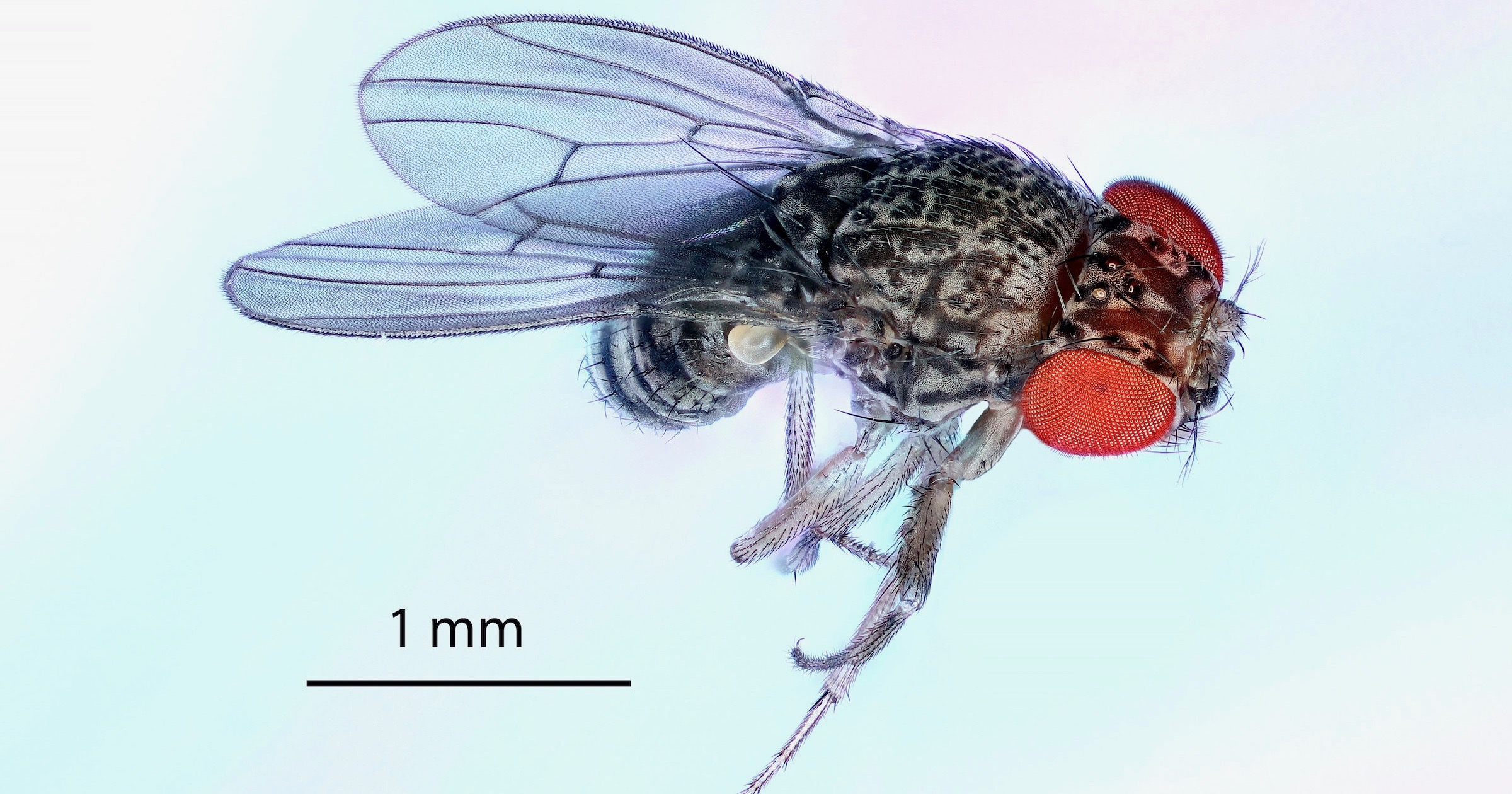
In previous articles, I summarized lectures at CELS (Conference on Engineering in Living Systems) that described two complementary engineering models for adaptation (here, here). This article will demonstrate how both models have been validated by studies on adaptation of insect wings.
Operational Gravity Well Model
The first engineering model presupposes that a biological structure or system is organized according to a specific design logic, architecture, or plan founded on engineering principles. The plan includes central features that are constant and unalterable, and it includes parameters that can vary within set limits. The model depicts the variation for traits in a population as an operational gravity well:
The allowed variation in an organism can be depicted as a series of variable operational parameters for the organism’s traits that, when taken together, can in turn be depicted as a three-dimensional space within which an organism can survive.
The model predicts that the variation corresponds to the adjustable parameters. And these parameters correspond to variables (e.g., wing size and thickness) whose values are tightly bounded. Of key importance, the fundamental design logic always remains intact.
Preservation of Design Plan
These predictions have been confirmed observationally and experimentally in studies of insect wings. Alba et al.’s 2021 study analyzed the diversity in Drosophila (fruit fly) wings. The researchers employed a sophisticated analytical method to map the differences in wing structure between individual flies in a single population and between different populations. They found that the basic architecture of the wings never altered.
They explained the permanence of the underlying structure as resulting from “global constraints within the developmental program” that ensure similar outcomes. In other words, developmental processes prevent the wing structure from fundamentally altering when perturbed by mutations and environmental fluctuations. Complex mechanisms continuously compensate for such perturbations to keep the embryo’s development on course.
Some mutations sufficiently impact development that corrective mechanisms cannot maintain the core design architecture. Such “macromutations” are invariably debilitating if not fatal. Their negative consequences further confirm the prediction of the gravity well model that any alteration of parameters beyond the predefined design constraints results in system failure.
Constrained Variability
In addition, the researchers linked the minor variation that did exist to “very weak mutations” and to phenotypic plasticity. Examples of the latter include flies developing modified wings in response to changing temperature and diet. Remarkably, the team was able to map the variation from both sources to differences in the value of a single variable that primarily corresponds to the precise locations where wing veins intersect.
Alba et al. summarize their work as follows:
Remarkably, we find that the outcomes of wing development can be statistically described by a one-dimensional (1D) linear manifold in morphological space that corresponds to a non-intuitive combination of structural variations across the wing. This dominant mode is systematically excited by variants generated by very weak mutations in signaling pathway genes as well as by thermal and dietary environmental perturbations. As such, our work provides direct empirical evidence for the presence of global constraints within the developmental program of the wing, funneling environmental inputs and genetic variation into phenotypes stretched along a single axis in morphological space. [Emphasis added.]
These observations perfectly match the predictions of the gravity well model that variation only exists for predefined adjustable parameters, and the values of related variables (e.g., locations of wing vein intersections) are tightly bounded.
Natural Genetic Engineering
Other research validates the tracking model for adaptation. The genetic differences corresponding to fly-wing variation are not primarily due to random mutations. Instead they resulted from natural genetic engineering (NGE). Up to 80 percent of “spontaneous” mutations in Drosophila are initiated by transposable elements (TEs). TEs are mobile segments of DNA that insert themselves into new regions of the genome. Of key importance, the relocation of TEs and their activation are often initiated by environmental stresses (here, here). And many insertions are targeted to specific locations (here, here). In the case of Drosophila, Weber et al. in a 2005 study identified multiple TE insertions that affect wing structure.
Investigators’ philosophical bias has led them to describe TEs using such terms as selfish, parasitic, or “invasive genetic elements” (here, here). These depictions contrast sharply with the extent to which they are often regulated and targeted and with the benefits they can confer to host organisms. Such observations are forcing the use of increasingly design-based language. Miller et al. in a 2021 review article about transposable elements and other NGE processes commented:
…biological variation is the product of collective differential assessment of ambiguous environmental cues by networking intelligent cells. Such concerted action is enabled by non-random natural genomic editing in response to epigenetic impacts and environmental stresses. Random genetic activity can be either constrained or deployed as a ‘harnessing of stochasticity’. Therefore, genes are cellular tools.
The phrase “harnessing of stochasticity” refers to cells initiating mutations or other semi-random genetic changes in specific DNA regions to create targeted genetic variation. This increase in diversity allows a population to fine-tune specific traits (e.g., insect wing structure) to best perform in different environmental conditions.
Phenotypic Plasticity
Multiple studies have also identified in insect wings examples of phenotypic plasticity. For instance, Bitner-Mathe ́and Klaczko in a 1999 study demonstrated that Drosophila respond to changing temperature and population density by altering their wing size, shape, and vein pattern. Fraimout et al. in a 2018 study described how Drosophila respond to lower temperatures by increasing the ratio of wing size to body size. The reduction in load on the wings compensates for cold-induced lower muscle efficiency. They also described how flies alter the shape of their wings to increase the stroke force to compensate for lower beat frequency.
Gao et al. in a 2019 study detailed an even more dramatic example in planthoppers. These insects track environmental cues such as temperature, food quality, and population density. Depending on the conditions, they can generate two different versions (aka phenotypes or morphs). In nutrient-rich environments, nymphs develop into the “short-winged” phenotype with reduced or missing wings, decreased flight muscle tissue, and lower energy metabolism. This form is more efficient in a local environment with abundant food. More importantly, this phenotype reproduces earlier and produces more eggs, which benefit the population in such favorable conditions.
The “long-winged” phenotype is generated if the environmental conditions deteriorate due to reduced food supply or overpopulation. This version has a well-developed flight apparatus with longer wings, larger flight muscles, and an enhanced energy metabolism. It can escape the unfavorable environment and colonize new locales.
The switching mechanism between the phenotypes is exceedingly intricate. Environmental sensors feed readings to the IIS signaling pathways that are commonly employed in complex organisms to control tissue growth and body size. This component triggers a host of other mechanisms that direct hundreds of genes including the production of alternative versions of proteins. The ingenuity and complexity behind this and the other referenced mechanisms not only highlight the extraordinary design behind biological adaptation, but they also reinforce the need for engineering models, tools, and language to broaden and expand our understanding of living systems.