Evolution
Intelligent Design
Engineering Better Explains Adaptation than Evolutionary Theory
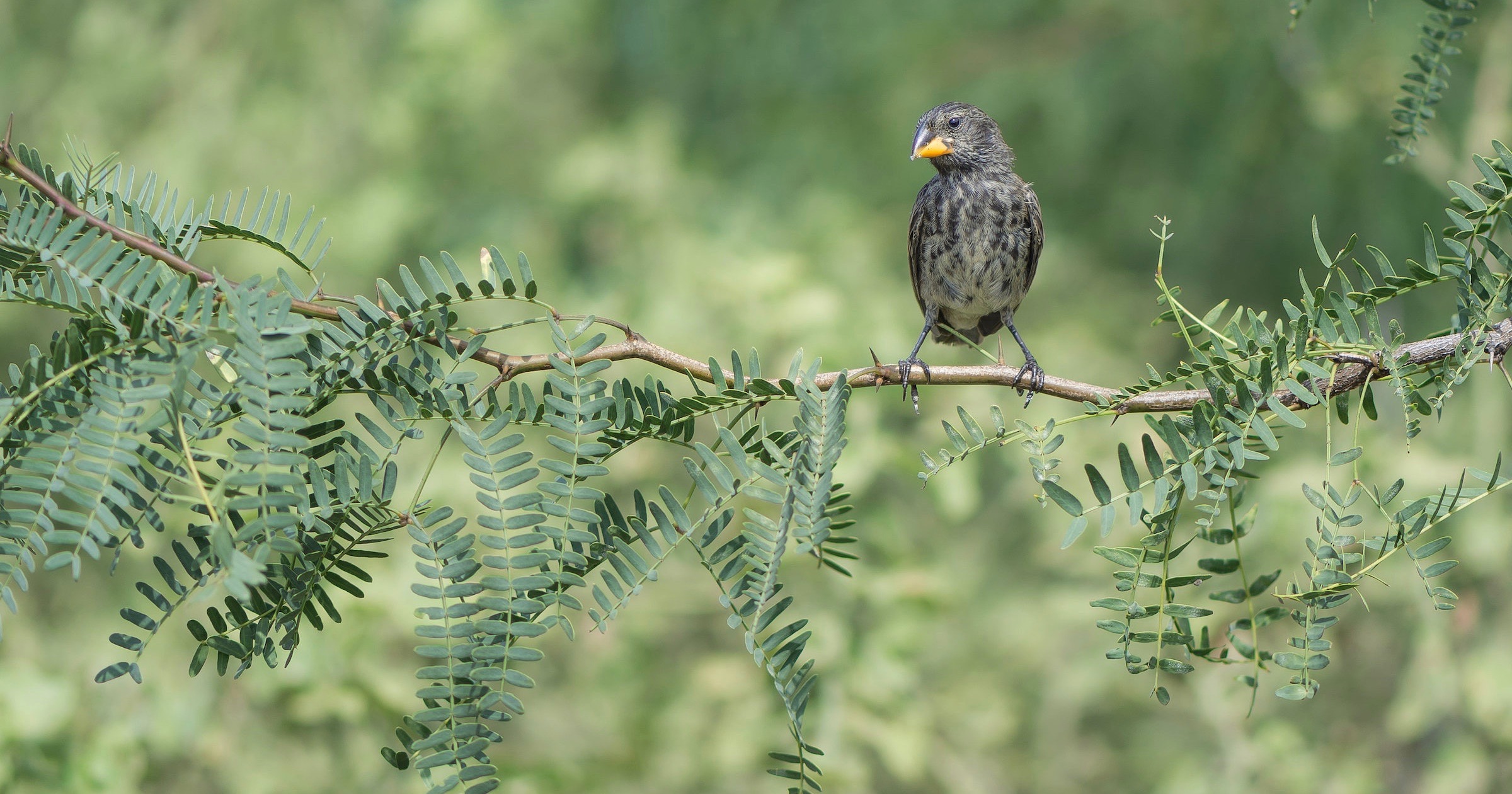
In recent articles, I have summarized how the field of systems biology has replaced evolutionary assumptions with design-based assumptions, language, and tools (here, here, here, here). The content drew from a lecture presented at the Conference on Engineering in Living Systems (CELS 2021). Now I will summarize another CELS lecture that demonstrated how biological adaptation is better explained by engineering principles than by evolution.
Comparing Models
The evolutionary model for adaptation is often depicted as a fitness landscape where the x-axis and the y-axis represent variation in traits (e.g., height, fur color) or genes (aka genotype), and the z-axis represents the likelihood an individual will produce surviving offspring (aka fitness). Each point along the landscape represents a particular combination of traits, and the dotted lines represent potential paths that a population could follow as it evolves across the landscape.
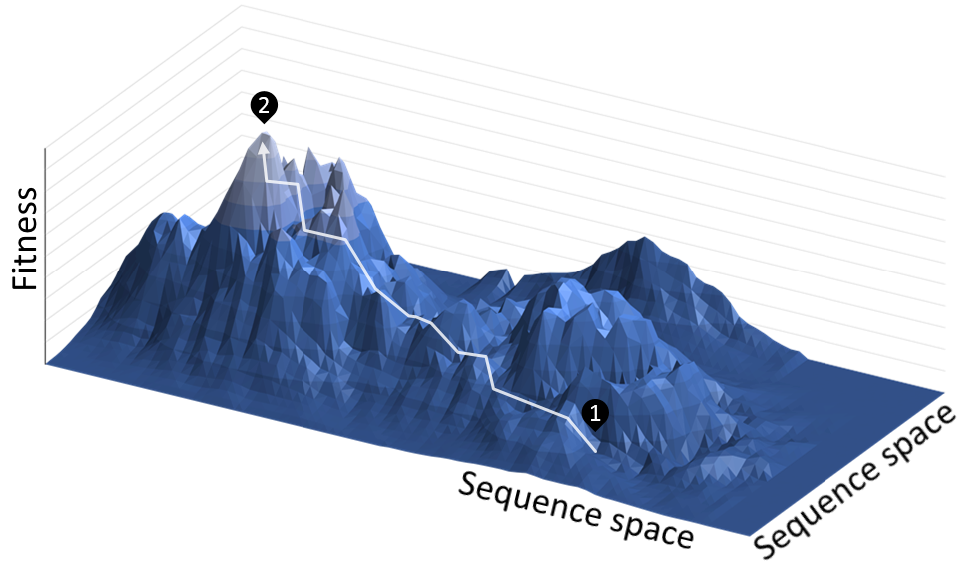
Mutations expand the variation, and natural selection is thought to drive the population to traverse the landscape, eventually resulting in large-scale changes. Since the peaks represent fitness optima, the trajectory of change is biased toward moving uphill, as natural selection drives the population toward greater fitness. Of particular importance, under the traditional evolutionary model the external environment drives adaptation, and the extent to which an organism can change is essentially unbounded.
In stark contrast, the engineering model argues that organisms are designed to operate within specific parameters determined by the overarching design logic. These parameters correspond to the extent to which the values of variables associated with an organism’s traits (e.g., thickness of a bone) can vary without system failure. An example of failure might include breaking a bone if the strain exceeds the operational range.
The Operational Well
The allowed variation in an organism can be depicted as a series of variable operational parameters for the organism’s traits that, when taken together, can in turn be depicted as a three-dimensional space within which an organism can survive. For example, a finch’s beak can properly function within a specific range of thickness (one variable) and within a specific range of length (another variable), and so on. In simplified form, these operating parameters can be represented as an operational gravity well. The center of the well represents optimal operating values associated with normal environmental conditions. The edge of the well represents the most extreme values that traits can tolerate before failure.
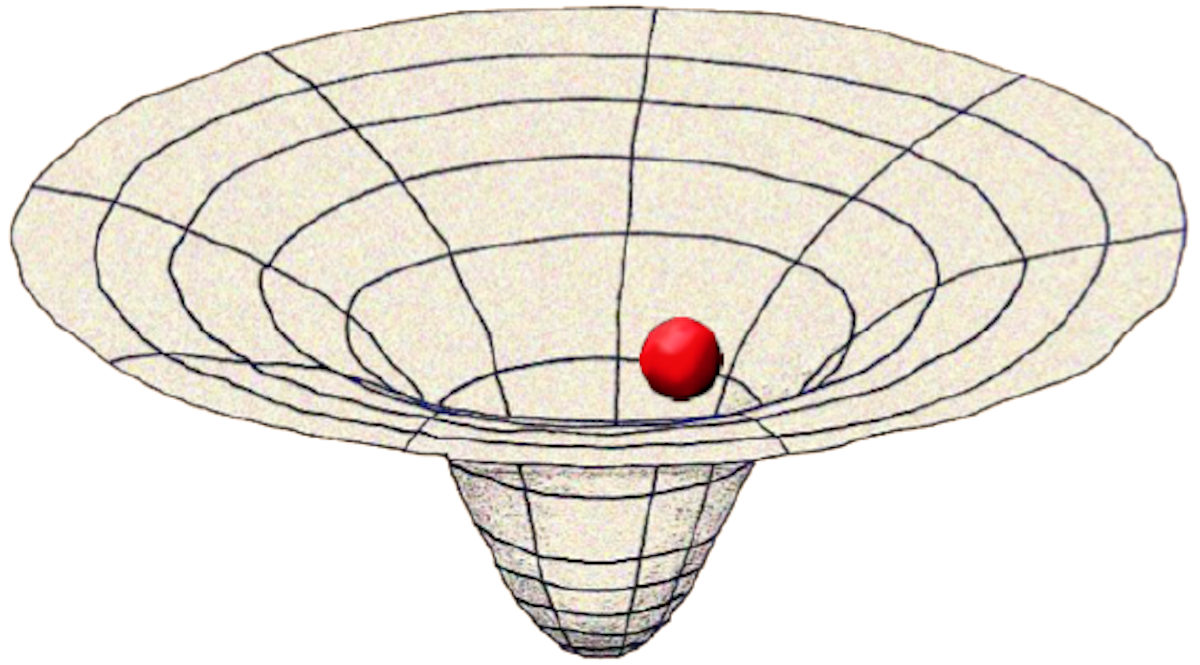
While environmental circumstances of course play a role in determining the extreme range of survivable conditions, it is the organism’s internal operating parameters that ultimately determine whether the organism can survive within a given environment. A lack of available food would require muscles to be maintained at a reduced size, but the muscles can only shrink by so much before a functional tolerance is exceeded. After that point, an animal could no longer move quickly enough to capture food or flee predators. It would then soon perish.
The variation in values is determined in part by the internal capacities of an individual to alter variables. For instance, an animal forced to place high stress on its limbs can often increase the strength of bones through internal adaptive mechanisms. Variation can also be determined by the genetic diversity in the population, whether originating/expanding through random mutations or through natural genetic engineering. Of particular importance, the operational parameters are not determined by the environment, but they are set by the design logic and established during construction. Moreover, the allowed variation is bounded.
The Hard Data
The empirical data consistently affirms the engineering model. The genetic variation in any species is confined to a limited set of variables such as a finch beak’s thickness. No genetic variation has been identified that would allow for the basic design logic and operational parameters to change. The average thickness of beaks may increase or decrease, but such changes always remain within a fixed range. And, more fundamentally, beaks always remain beaks.
No evidence has been identified that any trait in any species has started to transform into something fundamentally different. And no nonharmful mutation has ever been observed that would expand the genetic variation to allow such a change to occur in the future (here, here).
This conclusion is reinforced by the fossil record. Where the evidence is most abundant and clear, species are only observed to change during their tenure on Earth within the constraints of the operational parameters associated with their design logic. Anytime a new species appears with a new logic/architecture, it always appears abruptly without transitional forms leading back to a fundamentally different ancestor (here, here, here).
Future Research
The engineering model for adaptation not only better fits the empirical data, but it can drive more fruitful research. Infectious disease experts can identify the engineering principles behind pathogens and then better determine the limits of their adaptive capacities. This knowledge can lead to better vaccines and treatments. Biologists working with engineers can analyze living systems’ design logic and parameters. The resulting insights can help guide biomimetics research to craft better artificial limbs, nanomachines, micro air vehicles, and countless other innovations. And medical professionals can study the engineering of human anatomy and physiology to better understand the underlying operational constraints, leading to better treatments and preventative care. The possibilities are endless.
By recognizing that organisms are sophisticated entities designed with specific operational parameters and capabilities, an engineering-centered approach can contribute significantly to the growing field of systems biology, and it can provide additional insight into the remarkable biological systems in the world around us.