Intelligent Design
Life Sciences
Nearly All of Evolution Is Best Explained by Engineering
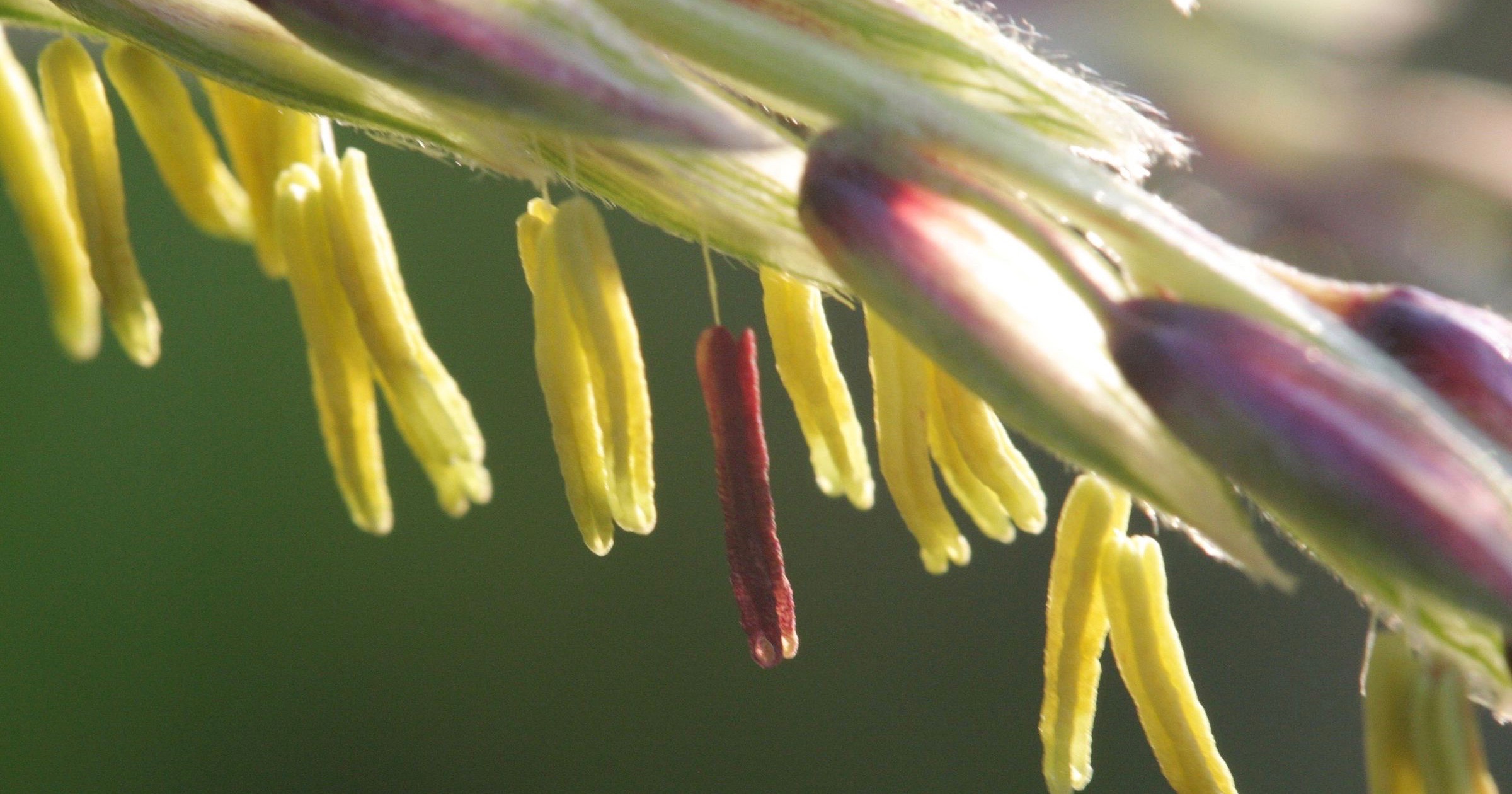
In recent articles, I have summarized lectures at CELS (Conference on Engineering in Living Systems) that described an engineering model for adaptation and explained how adaptation derives from organisms’ internal capacities (here, link). Now I will summarize another CELS lecture that expanded upon these themes by outlining a second complementary engineering model for adaptation.
Comparing Models
Standard evolutionary theory assumes that genetic variation expands through DNA mutating or otherwise altering randomly. Concurrently, natural selection and other processes transform species over time gradually through numerous, successive, slight modifications. The results are unpredictable, and in different subpopulations they can vary greatly.
In stark contrast, the presented engineering-based model assumes that organisms adapt to the environment using the same engineering principles seen in human tracking systems (here, here). More specifically, they continuously monitor the environment and track pre-specified environmental conditions. When the right conditions occur, internal mechanisms induce pre-determined responses such as targeted genetic changes, physiological adjustments, and/or anatomical alterations. These adaptive processes are directed by irreducibly complex systems that consistently include three components:
- Sensors to detect pre-specified environmental conditions such as temperature.
- Logic-based analyzers that determine if specific criteria are met such as the temperature exceeding a set point. When criteria are met, the analyzers send signals to trigger the appropriate responses.
- Processes that generate predetermined output responses when triggered, such as growing thinner hair.
The resulting changes are targeted, rapid, and often reversible. They are also predictable and repeatable. And their magnitude can range from minor alterations to dramatic transformations, but changes are bounded and predefined.
Over the past few decades, every facet of the engineering model has been increasingly affirmed by everyone from mainstream biologists to third-wave evolutionists to leading creationists (here, here, here, here). The strongest supportive evidence comes from studies of what have been termed natural genetic engineering (NGE) and phenotypic plasticity.
Natural Genetic Engineering
NGE refers to genetic alterations that are not random. Instead, they result from cells employing highly complex machinery to direct targeted DNA modifications. Leading researcher James Shapiro describes the processes in a 2016 review article:
Combinatorial coding, plus the biochemical abilities cells possess to rearrange DNA molecules, constitute a powerful toolbox for adaptive genome rewriting. That is, cells possess “Read–Write Genomes” they alter by numerous biochemical processes capable of rapidly restructuring cellular DNA molecules. Rather than viewing genome evolution as a series of accidental modifications, we can now study it as a complex biological process of active self-modification.
He further elaborates on the editing systems in a 2017 review article:
Like all classes of cellular biochemistry, NGE DNA transport and restructuring functions are subject to control by regulatory circuits and respond to changing conditions…NGE activities typically affect multiple characters of the variant cell and organism. Consequently, major phenotypic transformations can occur in a single evolutionary episode and are not restricted to a gradual accumulation of ‘numerous, successive, slight modifications.’
One could contest Shapiro’s claims about what NGE accomplished in the past, but his general description clearly matches the engineering model’s central features. The regulatory circuits that respond to environmental conditions correspond to sensors integrated with logic mechanisms. And the transport and restructuring functions correspond to specified output responses. In addition, the DNA modifications are targeted, rapid, and bounded as the engineering model expects.
NGE has been identified in all domains of life from the simplest to the most complex. Yeast cells respond to nutrient starvation by increasing the mutation rates at specific locations referred to as mutational hot spots. And the remarkable diversity in dog breeds is not the result of completely random mutations, but it also results from mutational hot spots that allow for increases in targeted genetic variation that can drive rapid adaptation. Biophysicists John Fondon and Harold Garner noted:
The high frequency and incremental effects of repeat length mutations provide molecular explanations for swift, yet topologically conservative morphological evolution…We hypothesize that gene-associated tandem repeats function as facilitators of evolution, providing abundant, robust variation and thus enabling extremely rapid evolution of new forms.
Equally striking, plant genomes contain DNA segments known as transposable elements (TEs) that can move to new locations, allowing them to alter the activity of local genes. Specific environmental stimuli can initiate relocation to target locations (here, here), and stimuli can activate the TEs, resulting in adaptive benefits. For instance, TEs modify gene regulation in maize to confer drought tolerance, alter flowering time, and enable plants to grow in toxic aluminum soils (here, here).
Phenotypic Plasticity
Phenotypic plasticity refers to an organism’s ability to transform its anatomy and physiology in response to environmental stimuli. The changes do not result from genetic alterations but from internal adaptive mechanisms. Developmental biologist Ralf Sommer enumerated these mechanisms’ essential components in a 2020 review article:
…plasticity requires developmental reprogramming in the form of developmental switches that can incorporate environmental information. However, the associated molecular mechanisms are complicated, involving complex loci, such as eud-1, that function as switches and GRNs. While still early, it is likely that switch genes point to a general principle of plasticity because other examples of plasticity also involve complex switch mechanisms.
The “incorporation of environmental information” tacitly implies the presence of sensors and signal transmission pathways. The switch incorporating the sensory output equates to a logic-based analyzer, and the gene regulatory network (GRN) activity corresponds to the output response. In summary, the core components perfectly match those of the engineering model for adaptation.
Phenotypic plasticity has been observed in numerous species in diverse taxa. Gulls of the family Laridae track the sodium level in their blood with sensors in heart vessels. When the level reaches a threshold, gulls generate a specialized gland that extracts excess sodium from the blood and excretes it through the beak. If the gull migrates to a freshwater environment, the gland disappears.
Cichlid fish demonstrate phenotypic plasticity for multiple traits. Muschick et al. in a 2011 study raised Midas cichlids on food with different hardnesses. The different diet groups developed significantly different pharyngeal jawbones, and the differences resembled qualitatively the differences in jawbones found in specialized species. Härer et al. in a 2019 study exposed Midas cichlids to light of different frequencies. In response to a change in frequency, the cichlids switched the expression of cone opsin genes crucial for color vision in only a few days. Other such mechanisms likely exist, based on the observation that cichlids rapidly converge to the same basic forms repeatedly.
As a final example, fish residing in cave environments display distinctive traits such as reduced eyes and pigmentation. The standard evolutionary story is that these traits gradually developed through natural selection. But experiments over the past decade on the effects of exposing fish to cave-like conditions are changing the narrative.
Rohner et al. in a 2013 study raised A. mexicanus embryos in water with low conductivity mimicking cave conditions. The embryos developed into adults with significantly smaller eyes. Corral and Aguirre in a 2019 study raised A. mexicanus in different temperatures and different levels of water turbulence. The variant conditions resulted in adult fish differing in vertebral number and body shape. For instance, fish raised in more turbulent water displayed more streamlined bodies and extended dorsal and anal fin bases that improved their mobility in that environmental condition. And Bilandžija et al. in a 2020 study raised the same species in darkness, and the fish developed many cave-related traits such as resistance to starvation and altered metabolism and hormone levels. Future research will likely uncover even more examples where cave-specific adaptations result not from random mutations but from internal mechanisms.
Future Research
The engineering model not only best fits the latest experimental and observational data, but it can help guide future research. Whenever a species rapidly and predictably adapts to a specific environmental condition (here, here, here), investigators can expect that changes are directed by sensors, logic-based analyzers, and output response mechanisms. They can then focus research on identifying and understanding these components.
Traditional evolutionary processes do play a part in biological adaptation, but mounting evidence demonstrates that their role is relatively minor in the drama of life (here, here). Instead, engineered adaptive mechanisms that direct targeted modifications perform on center stage.