Evolution
Intelligent Design
Origin of Life: Top Three Problems with Protocells
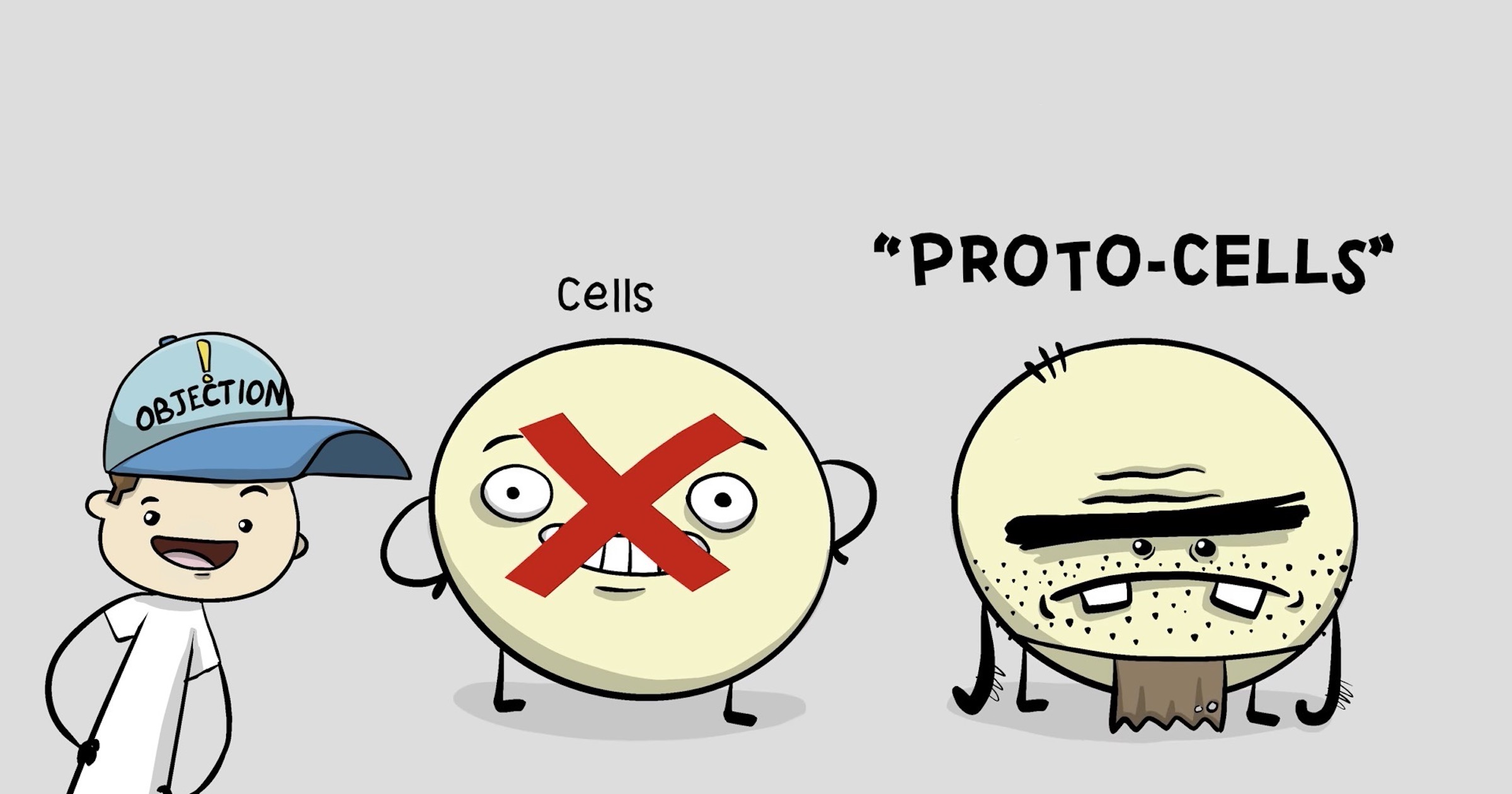
The latest video in the Long Story Short series was released this week on YouTube. The video explains how cell membranes in all of life display complexity that cannot be explained by purely natural processes. See my comments from yesterday, “New Animated Video: Cell Membranes by Natural Processes Alone?,” adding some supporting details to the argument. Here’s more.
As we uncover layer after layer of the astounding complexity of even the simplest forms of life, the origin-of-life research community increasingly relies upon their trump card: imaginary protocells that supposedly existed long ago and were dramatically simpler than existing life. As the story goes, modern life may indeed be very complex, but protocells used to be much simpler, and there was plenty of time for the complexity to develop.
Protocells conveniently fill the uncomfortably large gap between the simple molecules that can be produced by prebiotic processes and the staggering complexity of all extant life. But there are three major problems with the concept of protocells. These problems are all backed by strong empirical support, in sharp contrast with the concept of protocells.
A Coddling Environment
First, scientists have been working for decades to simplify existing life, trying to arrive at a minimal viable life form by jettisoning anything that is not essential from the simplest extant cells. The success of Craig Venter’s group is well known. Building on their efforts to produce synthetic life (“Synthia” or “Mycoplasma labritorium”) in 2010,1,2 in 2016 they introduced the current record holder for the simplest autonomously reproducing cell (JVCI Syn3.0).3 With a genome of only 473 genes and 520,000 base pairs of DNA, JVCI Syn3.0 can reproduce autonomously, but it certainly isn’t robust. Keeping it alive requires a coddling environment — essentially a life-support system. To arrive at a slightly more stable and robust organism that reproduced faster, the team later added back 19 genes to arrive at JVCI Syn3A.4 When combined, this work provides an approximate boundary for the simplest possible self-replicating life. We are clearly approaching the limit of viable cell simplicity. It seems safe to conclude that at least 400 genes (and approximately 500,000 base pairs of DNA) are the minimum requirements to produce a self-replicating cell.
Exporting to the Environment
Second, we know that the process of simplifying an existing cell by removing some of its functionality doesn’t actually simplify the overall problem — it only exports the required complexity to the environment. A complex, robust cell can survive in changing conditions with varying food sources. A simplified cell becomes dependent on the environment to provide a constant, precise stream of the required nutrients. In other words, the simplified cell has reduced ability to maintain homeostasis, so the cell can only remain alive if the environment takes on the responsibility for homeostasis. Referring to JVCI Syn3A, Thornberg et al. conclude, “Unlike most organisms, which have synthesis pathways for most of [their] building blocks, Syn3A has been reduced to the point where it relies on having to transport them in.”5 This implies that the environment must provide a continuous supply of more specific and complex nutrients. The only energy source that JVCI Syn3A can process is glucose,4 so the environment must provide a continuous supply of its only tolerable food. Intelligent humans can provide such a coddling life-support environment, but a prebiotic Earth could not. Protocells would therefore place untenable requirements on their environment, and the requirements would have to be consistently met for millions of years.
Striving for Simplicity
Third, we know that existing microbes are constantly trying to simplify themselves, to the extent that their environment will allow. In Richard Lenski’s famous E. coli experiment, the bacteria simplified themselves by jettisoning their ribose operons after a few thousand generations, because they didn’t need to metabolize ribose and they could replicate 2 percent faster without it, providing a selective advantage.6 Furthermore, Kuo and Ochman studied the well-established preference of prokaryotes to minimize their own DNA, concluding: “deletions outweigh insertions by at least a factor of 10 in most prokaryotes.”7 This means that existing life has been trying from the very start to be as simple as possible. Therefore, it is likely that extant life has already reached something close to the simplest possible form, unless experimenters like Lenski provide a coddling environment for a long duration that allows further simplification. But such an environment requires the intervention of intelligent humans to provide just the right ingredients, at the right concentrations, and at the right time. No prebiotic environment could do this. Therefore, scientists need not try to simplify existing life — we already have good approximations of the simplest form. Indeed, Mycoplasma genitalium has a genome of 580,000 base pairs and 468 genes8 whereas Craig Venter’s minimal “synthetic cell” JVCI Syn3.0 has a comparable genome of 520,000 base pairs and 473 genes.3
The data provide a clear picture: the surprising complexity of even the simplest forms of existing life — 500,00 base pairs of DNA — cannot be avoided and cannot be reduced unless intelligent agents provide a complex life-support environment. Because protocells would have had to survive and reproduce on a harsh and otherwise lifeless planet, protocells are not a viable concept. Protocells place origin-of-life researchers in a rather awkward position: relying upon an imaginary entity to sustain their belief that only matter and energy exist.
References
- Gibson DG et al. Creation of a bacterial cell controlled by a chemically synthesized genome. Science 2010; 329:52–56.
- Gibson DG et al. Synthetic Mycoplasma mycoides JCVI-syn1.0 clone sMmYCp235-1, complete sequence. 2010. NCBI Nucleotide. Identifier: CP002027.1.
- Hutchison CA III et al. Design and synthesis of a minimal bacterial genome. Science. 2016; 351: 1414.
- Breuer et al. eLife 2019; 8:e36842. DOI: https://doi.org/10.7554/eLife.36842.
- Thornburg ZR et al. Fundamental behaviors emerge from simulations of a living minimal cell. Cell 2022; 185: 345-360.
- Cooper VS et al. Mechanisms causing rapid and parallel loss of ribose catabolism in evolving populations of Escherichia coli B. J Bacteriology 2001, 2834-2841.
- Kuo, CH and Ochman H. Deletional bias across the three domains of life. Genome. Biol. Evol. 1:145–152.
- Fraser CM et al. The minimal gene complement of Mycoplasma genitalium. Science. 1995; 270; 397-403.