Intelligent Design
Physics, Earth & Space
In First Detection of Gravitational Waves, Timing Was Everything
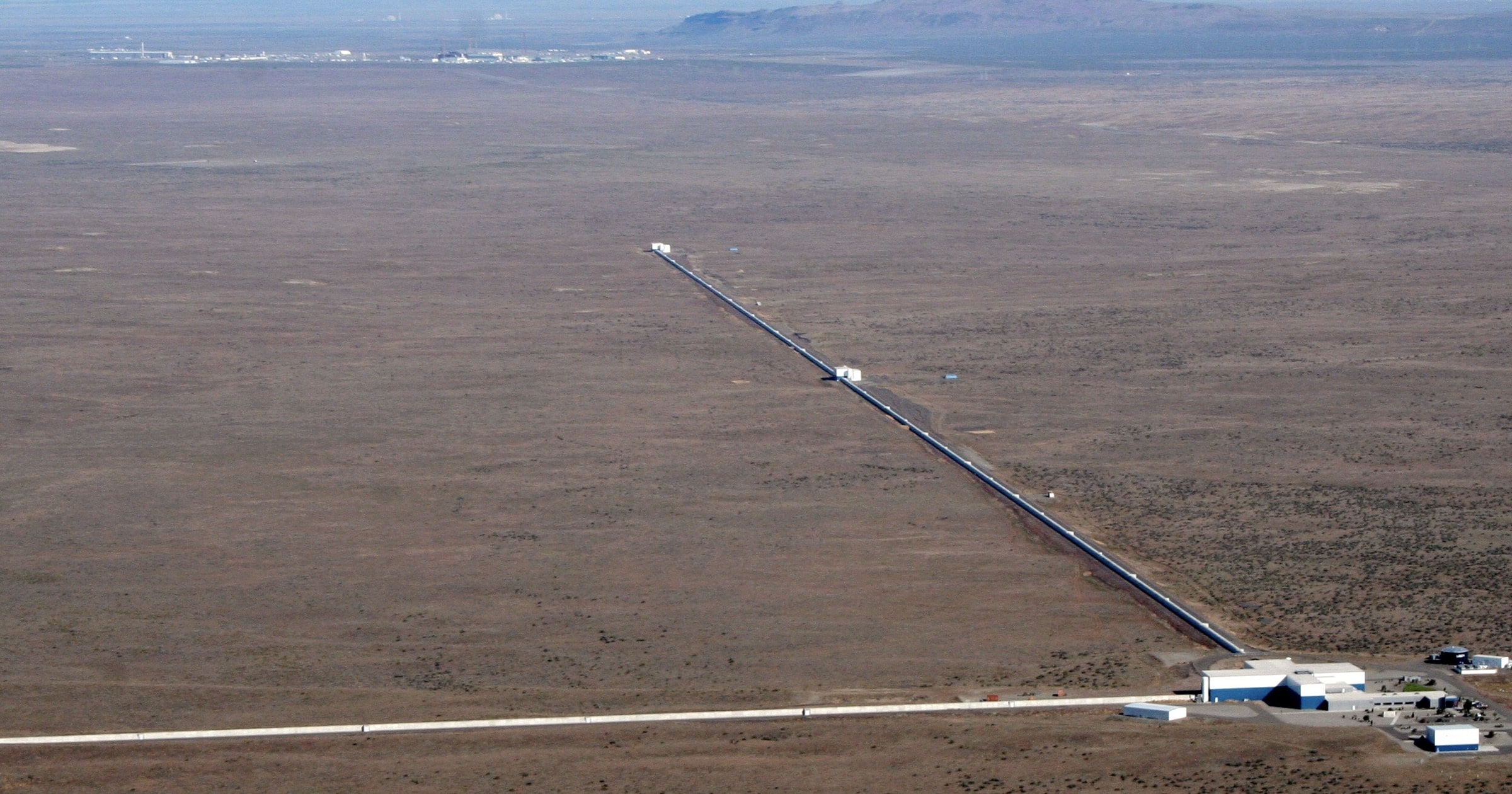
Heralding one of the most significant scientific discoveries in the last hundred years, scientists announced in early 2016 the first-ever direct detection of a gravitational wave. Both the wave and its source — massive black holes formed as the collapsed remnants of giant stars — are stunning predictions of Einstein’s general theory of relativity.
The prediction and subsequent detection of gravitational waves highlights both the fine-tuning of the universe for discoverability and human exceptionalism that extends with these achievements far beyond any abilities that would be expected through a putative evolutionary origin of our race.
These events also reveal an aspect of evidence for design that some have termed fine-timing (see here and here). Let’s look at how timing across multiple orders of magnitude brought together cosmic and human events in the first-ever detection of a light speed that was non-electromagnetic in nature.
Like a Seismic Wave
Gravitational waves act like a seismic wave propagating through space that manifests as a shaking of the spacetime fabric of the heavens. When we consider the temporal details of events leading to the first detection of a gravitational wave — time frames that range from a fraction of a second to a span of years covering nearly the entire age of the universe — we see that timing is everything.
In a distant era when our universe was much younger than today, two unusually massive stars, birthed into an ever-circling orbital dance, filled surrounding space with torrents of electromagnetic energy. Estimates of the masses of these ancient stellar giants dwarf our sun in comparison — 60 to 70 times the mass of the sun. Gravity, the antagonist of this stellar drama, is initially withstood by the internal pressure of a commensurately powerful nuclear furnace raging within the core of each star.
Such a prodigious output of energy, required to withstand gravity’s relentless crushing force, comes at a cost, and the massive stars’ nuclear furnaces run out of fuel a thousand times sooner than the lifespan allotted to our milder sun. Their finale comes with a dramatic transformation as the two stars, one after another, lose their battle against gravity and collapse into the ultimate negation of a star of light — a black hole.1 Now the two, once-luminous giants continue their dance in the blackness of non-light, their masses having retreated into the abyssal depths of over-warped spacetime — all that remains of the former brilliant pair.
In silence and darkness, the massive black holes continued their binary orbit in a distant galaxy 1.3 billion lightyears away. They emit no light — the inverted fortress of gravity has become so strong that no radiation of any wavelength can escape these wells of darkness. And yet, their unending motion constantly sends whispering ripples throughout the black pond of surrounding space.
Back on Planet Earth
Far away on Earth in the year 1916, about 11.3 billion years after the black holes swallowed their natal stars, a brilliant scientist formulates a remarkable theory. Einstein, in his general theory of relativity, describes how gravity results from matter causing a warping of space and time, or “spacetime.”
Einstein’s theory also predicts the emanation of gravitational waves through space from the accelerated motion of matter within the fabric of space, much as waves ripple across a lake from a rock thrown into the water.
Over the course of time, the gravitational waves emanating from the orbiting black hole pair steadily deplete energy from the system, causing them to revolve ever closer together. Like water swirling down a drain, the orbiting black holes move faster and faster as they fall inward toward one another. In the last tenth of a second before the inspiraling black holes merge together, their orbital velocity reaches half the speed of light, and a sudden crescendo of spacetime vibrations rings forth.2 Remarkably, this powerful burst of gravitational wave energy momentarily eclipses the combined power output of the entire universe as it reverberates throughout surrounding space like the powerful blow of a hammer on a gong.3
To detect gravitational waves requires an entirely different approach from detecting light waves. The measurable effect of a passing gravitational wave comes from how it shifts one point in space with respect to another point. For example, a gravitational wave would cause the length of a meter stick to alternately become slightly longer and then shorter as the wave oscillated past. The difficulty in detecting gravitational waves emitted a billion light years away is that the change in length would typically be far less than the size of the smallest sub-atomic particle.
The LIGO Interferometer
Undeterred by this challenge, scientists designed the Laser Interferometer Gravitational-Wave Observatory (LIGO), capable of detecting such minute changes in length. The LIGO interferometer consists of two perpendicular arms, each 4 km long, with powerful laser beams continuously reflecting back and forth along their lengths. Mirrors and detectors at the ends of the long arms are so sensitively aligned and balanced that they can register a shift in the arms’ lengths of only one ten-thousandth of the diameter of a single proton.4
Two such detectors were commissioned, one in Hanford, Washington, and another in Livingston, Louisiana. The initial LIGO observation run covered the first decade of the 2000s, during which time it failed to detect any gravitational wave signals from space. Then scientists scheduled a break in its operation while the instruments underwent a major upgrade to provide LIGO with ten times more sensitivity than before.
After seven years of preparation, the advanced LIGO facility began its first observing run in September 2015, and just days after it became fully operational the gravitational wave pulse from the final coalescence of the black hole pair arrived at Earth, hitting the two advanced LIGO instruments a mere 7 milliseconds apart.2, 5
After having travelled through space at the speed of light for 1.3 billion years from its far-off galaxy of origin, this reverberation of the heavens reached Earth just days after humans were first equipped to capture its now faint signature. The momentous detection of this incredible signal culminated 100 years of prediction, speculation, and searching that began with Einstein’s solutions of his general relativity equations in 1916, indicating that such waves should exist.
The structure and duration of the detected signals matched the predicted signature of a gravitational wave produced by the inward spiraling and merger of two massive black holes, rapidly orbiting each other in a distant galaxy. Theoretical analysis of the gravitational wave signals revealed that the original black hole masses were 36 and 29 times the mass of our sun, or almost twice as big as the most massive stellar black hole previously known.7 In the final moment of their coalescence, approximately three solar masses of their combined mass were rapidly converted into the energy of the gravitational wave pulse. To put the power of this pulse into perspective, the energy equivalent of three solar masses is over 4,000 times the expected total energy output of our sun over its entire 10-billion-year lifespan as a main sequence star. And the furiously inspiraling black holes emitted this much energy in less than half a second!
More than Happenstance
The timing of these events suggests more than happenstance. Since the detectable pulse of the gravitational wave is so transient, if the advanced LIGO detectors had been delayed by just a couple of weeks in starting their observations, the wave would have passed by unnoticed.
When Einstein predicted gravitational waves as part of his general theory of relativity in 1916, the gravity wave pulse from the climactic inspiraling of the two black holes had approached to within 99.99999 percent of Earth. Much significant human history still had to unfold during the century from 1916 to late 2015, but from the point of view of the gravity wave, it was already on our doorstep, and would soon make itself evident to us.
The LIGO detection of the first gravitational wave from its incredibly distant source in the heavens was the smallest signal ever discerned by any scientific instrument in the history of mankind. In contrast, the origin of the wave pulse was one of the most powerful events ever produced in the history of our universe. Sometimes, the slightest whispers of discernment may reveal the most momentous events.
We have often felt the shaking of the Earth, but not until 2015 had we ever discerned the shaking of the heavens.
References
- K. Belcznski, D. E. Holz, T. Bulik, and R. O’Shaughnessy, “The First Gravitational-Wave Source from the Isolated Evolution of Two Stars in the 40-100 Solar Mass Range,” Nature, 534, 512 (2016).
- B. P. Abbott, et al, “Observation of Gravitational Waves from a Binary Black Hole Merger,” Phys. Rev. Lett. 116, 061102 (2016).
- 2017 Nobel Prize in Physics Awarded to LIGO Founders | LIGO Lab | Caltech.
- LIGO, https://www.ligo.caltech.edu/.
- B. P. Abbott, et al, “GW150914: The Advanced LIGO Detectors in the Era of First Discoveries,” Phys. Rev. Lett. 116, 131103 (2016).
- A. Buonanno, “Gravitational Wave Astronomy: The Dawn of a New Era,” CERN Courier, Jan./Feb. 2017, p. 17.
- https://en.wikipedia.org/wiki/M33_X-7.