Evolution
Intelligent Design
Sunlight on the Puzzle of Prebiotic RNA?
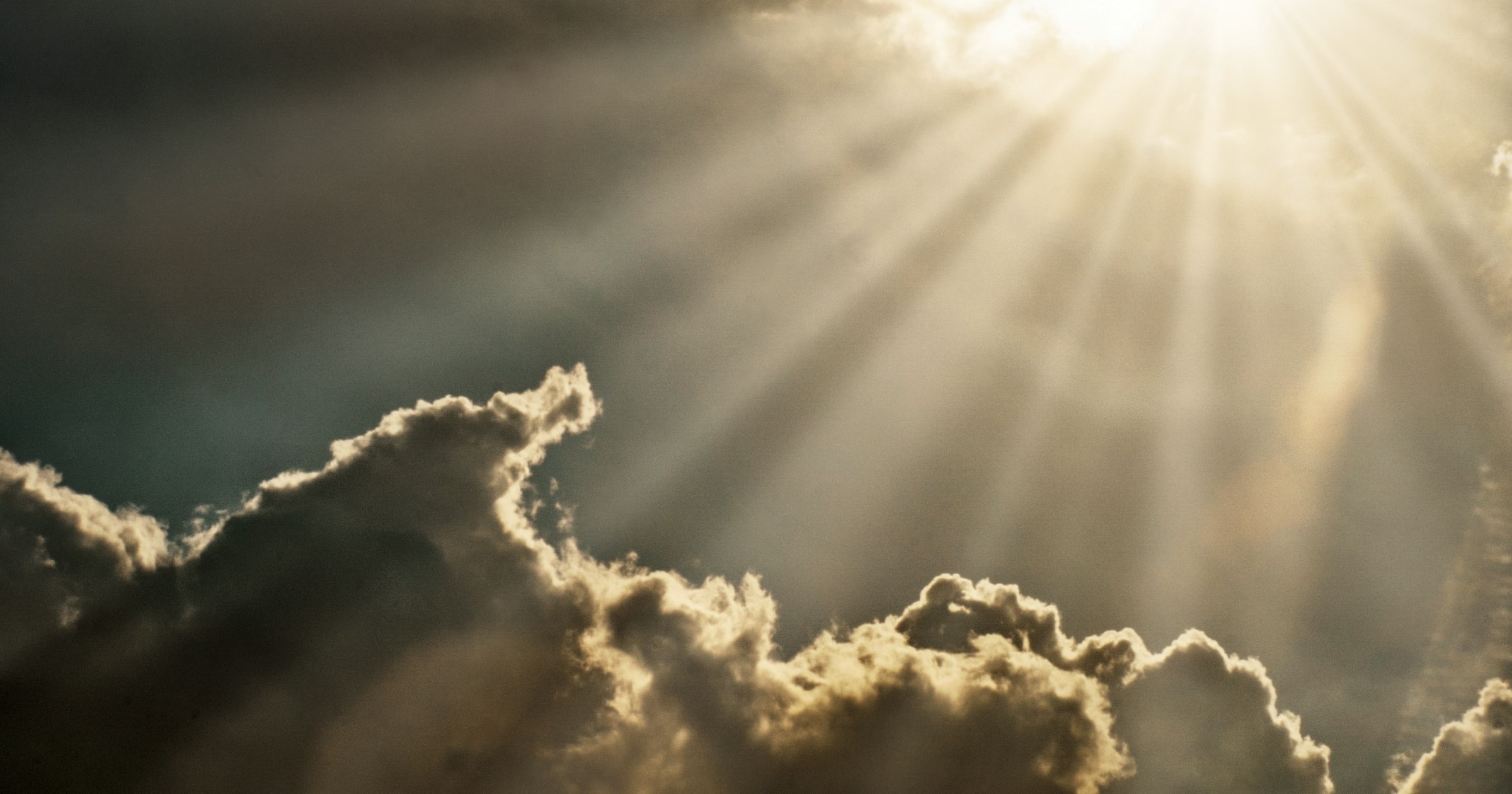
In two previous articles for Evolution News (here and here) I have argued against the plausibility of generating biologically relevant polymers (proteins, RNA, and DNA) in a prebiotic world. Much of this argument was based on the requirement for homochirality of the building blocks and the precise chemical bonds necessary to achieve nucleotides for RNA and DNA. Admittedly, some scientists have proposed schemes that are more palatable to skeptics of origin of life (OOL) research. Therefore, here I will explain the positive steps that have recently been taken to solve the enigma of how RNA and DNA may conceivably arise in an abiotic world.
In my earlier article, discussing the discovery of uracil recovered from samples taken from an asteroid, I made clear that formation of RNA (or DNA) building blocks through linking ring-structured nucleobases (uracil, thymine, cytosine, adenine, guanine) to the ring-constrained back bone sugar ribose (or deoxyribose for DNA) is a hopeless abiotic endeavor. The chemistry needed to complete formation of the needed building blocks in high yield without the use of energetically charged substrates and enzymes (as living systems use) is untenable. Several OOL scientists have recognized this drawback. They have chosen a different path, considered below, to propose how biologically relevant nucleotides might have been produced chemically, using only starting compounds believed to be present prebiotically.
Facile Synthesis of Pyrimidine Nucleosides (Cytidine and Uridine)
The chemical scheme envisaged employs a mechanism to build pyrimidine ring structures ab initio on ribose, rather than attempting to link them to this sugar. The entire synthetic process is as follows:
Disparate reaction conditions in the multi-step syntheses (conditions are in bold text):
Step 1) Ribose + cyanamide / 0.2 M sodium carbonate (pH ~10), 55o C -> RAO
Step 2) RAO + cyanoacetylene / hydrosulfide in aqueous formamide, UV light -> Intermediates A
Step 3) Intermediates A / phosphate, anhydrous formamide, heat -> alpha & beta-ribopyrimidines (pyrimidine ribonucleosides completed)
Step 4) Intermediates A + 8-mercaptoadenine / dry state in magnesium chloride, 150o C -> Intermediates B
Step 5) Intermediates B / bisulfate in phosphate (pH 7), 60o C or sulfite in phosphate (pH 8-10), heat, UV light -> Intermediates C
Step 6) Intermediates C / nitrous acid (pH 4) -> adenine and inosine products (purine ribo- and deoxyribonucleosides completed)
The first step uses cyanamide, a compound likely present on the early Earth, to react with ribose in a two-step process that traps the 5-carbon sugar into the required furanose ring conformation.1 This putative RNA precursor, called ribo-aminooxazoline (RAO), now has the first three atoms destined to form the pyrimidine ring already attached to the appropriate carbon of ribose. It is noteworthy that ribose, competing against the other three possible 5-carbon sugars, is the preferential substrate in this reaction. From this point synthesis continues2 via step 2 in the table, using another simple abiotic prospect, cyanoacetylene, resulting in an intermediate that is readily converted to ribocytidine. Thus, in just three steps using simple reactants, the first nucleoside is nearly complete in fairly high yield. This product, however, needs to be in the beta configuration. But this is easily managed by exposure to UV light with a conversion rate as high as 74 percent. UV light also facilitates oxidation of cytosine to uracil (at much lower yield) completing the recipe for production of both pyrimidine nucleosides. The authors in the publication cited above describe an alternative route to uridine, offering some advantages in yield by performing the reaction with cyanoacetylene in the presence of hydrosulfide.
Facile Synthesis of Purine [Deoxy]Nucleosides ([Deoxy]Adenosine and [Deoxy]Inosine)
To tackle the production of purine nucleotides, another putative chemical precursor feasibly present in an abiotic setting (step 4) is reacted in the presence of bisulfate with an intermediate in the alpha-ribocytidine pathway.3 Two isomers of beta-deoxyadenine (dA) result upon UV irradiation where the biologically relevant isomer exhibits yields close to 50 percent. Treatment of dA with nitrous acid gives partial conversion to beta-deoxyinosine (dI). Subsequent research by this group demonstrated the feasibility of producing the corresponding purine ribonucleosides (A and I) by carrying out the first reaction in alkaline sodium sulfite instead of bisulfate.4 The yields of the desired products are appreciably lower than those found for the pyrimidine products (10-40 percent vs 40-50 percent). In summary, these chemical synthetic studies under hypothetical prebiotic conditions have demonstrated respectable yields for the beta versions of ribo pyrimidines and purines as well as for beta versions of deoxyribo purines, representing the canonical building blocks used in biology.
The Homochirality Challenge
None of the studies indicated above differentiate between the two stereoisomers of ribose. Certainly, L-ribose should have been equally present with D-ribose in a prebiotic world, so RAO precursors would likewise be a racemic mixture. Another laboratory explored an innovative approach taking advantage of the fact that chiral molecules can align with a magnetic field due to spin states of all their electrons.5 Enantiomeric pairs of a compound will exhibit spin states aligning with opposing magnetic polarity. This principle is known as chiral-induced spin selectivity (CISS). Because magnetite is expected to be abundant in the sediment of bodies of water on the primordial Earth, it was hypothesized that Earth’s magnetic field would serve to magnetize the iron-containing sediment, providing a means to preferentially adsorb RAO enantiomers in different zones based on their CISS. The referenced experiments were performed in Petri dishes simulating this design, placing a magnet underneath the insoluble magnetite. The first round of selection eventually led to RAO crystallization on the magnetite surface, demonstrating a significant enrichment in either D- or L-RAO depending on magnetic polarity.
After collecting the crystals, redissolving them in buffer, and repeating the magnetite-induced selection, the second round of crystallization resulted in optically pure RAO. From experiments like these it was proposed that homochirality of RAO could have been achieved on the prebiotic Earth where a small enantiomeric imbalance is induced within zones to retain preferentially one of the two RAO stereoisomers. With this occurring near shore, water flow redissolves the RAO crystals, followed by a subsequent dry phase when the shoreline recedes. When the water rises to this level again, RAO recrystallizes to a greater enantiomeric excess. That is since the magnetite had been conditionally enhanced magnetically in the previous round through the CISS effect.6 It was suggested that this repeated cycle of adsorption, crystallization, and spin-alignment on the magnetite surface may serve as a positive feedback loop, leading to the ultimate prize of crystalizing homochiral RAO.
Feasibility of This Chemistry Was Demonstrated, but Is It Plausible Under Prebiotic Conditions?
Considering first the proposal to attain homochirality of RAO, weak assumptions are required to accept this achievement. The authors noted that timing of crystallization is important. That is because if crystals are allowed to grow for too long a period of time, enantiomeric excess decreases and thus the chiral spin state training of magnetite is reduced or jeopardized. It would take a string of good fortune to have that cycle spontaneously repeated at the right length of time. It would also be expected that different zones along the shore would each select their own enantiomer of RAO.6 Despite having individual homochiral crystals, over larger areas the opposite stereoisomers are also crystallized. So how do they remain separated before and after undergoing subsequent reactions, leading to nucleoside synthesis? This can be readily accomplished in the laboratory, keeping crystals and their down-stream products isolated. But there is no guarantee they will remain isolated under abiotic situations.
Regarding the synthesis of nucleosides from RAO, several concerns come into play: 1) Multi-step syntheses diminish the yields of final products and increase probabilities of generating by-products that might compete for incorporation into RNA building blocks. The yields of purine nucleosides, in particular, suffer from the requirement for additional steps needed in their synthesis. 2) Most reactions require disparate conditions. How do the reactants in an abiotic environment become sequentially incorporated under these different reaction conditions (Steps 1-6)? In the laboratory this is self-explanatory, but it is not a trivial matter when chemistry is left to stochastic events. 3) Predictions of the chemical repertoire of a prebiotic Earth are largely unsubstantiated. Support for such predictions could potentially come from analyzing samples from other extraterrestrial bodies as was done with the asteroid Ryugu. Retrieval of samples from Mars would be a particularly exciting venue to explore. Without this type of confirmatory data our suppositions of prebiotic chemistry could simply be shooting in the dark. 4) The role of UV light is likely critical to the formation of these RNA building blocks. This can be a double-edged sword as UV light can also damage purines and pyrimidines by several means. 5) A prebiotic synthetic scheme leading to guanine has still not been elaborated. Instead, they propose that inosine, which is included in the schemes presented here, can substitute for guanine since it is able to base pair with cytosine (as guanine does). Inosine, however, is more akin to being a wildcard as it also base pairs with uracil and thymine. Such promiscuous base pairing will undoubtedly limit the functional gamut of RNA/DNA polymers and alter the structural dynamics that we now know are important for the biological roles of many RNAs.
Truly an Extraordinary Solution
I appreciate the quality of work that went into devising chemical schemes to synthesize purine and pyrimidine nucleobases, achieving the mandatory stereo and chemical specificity of furanosyl ribose established in living systems. This is truly an extraordinary solution using alleged prebiotic conditions. In my opinion, other attempts to meet this challenge have not come close to attaining such a level of accuracy. Unfortunately, the most significant problem is how this can be completed without a guided mechanism to direct each successive reaction and maintain respectable yields along the entire process.
Beyond the initial synthesis of nucleic acid building blocks, there are more highly significant concerns once nucleotide chains have been assembled. Adjacent pyrimidines are very prone to form several types of chemical lesions elicited by UV light. Without a series of enzymes to repair such lesions, the repercussions for the damaged RNA/DNA molecule are poor. In current biological systems they result in mutations because of replication errors. Of course, in a prebiotic world repair enzymes would not exist. These concluding remarks shine light (pardon the pun) on future considerations of RNA/DNA polymers in a prebiotic world. If one wonders why origin-of-life science has its skeptics, unpretentious complications like those here reveal concerns and doubts that arise as we contemplate the field.
References
- Springsteen G, Joyce GF. Selective derivatization and sequestration of ribose from a prebiotic mix. J Am Chem Soc 2004;126(31):9578-83 doi 10.1021/ja0483692.
- Xu J, Tsanakopoulou M, Magnani CJ, Szabla R, Sponer JE, Sponer J, et al. A prebiotically plausible synthesis of pyrimidine beta-ribonucleosides and their phosphate derivatives involving photoanomerization. Nat Chem 2017;9(4):303-9 doi 10.1038/nchem.2664.
- Xu J, Chmela V, Green NJ, Russell DA, Janicki MJ, Gora RW, et al. Selective prebiotic formation of RNA pyrimidine and DNA purine nucleosides. Nature 2020;582(7810):60-6 doi 10.1038/s41586-020-2330-9.
- Xu J, Green NJ, Russell DA, Liu Z, Sutherland JD. Prebiotic Photochemical Coproduction of Purine Ribo- and Deoxyribonucleosides. J Am Chem Soc 2021;143(36):14482-6 doi 10.1021/jacs.1c07403.
- Ozturk SF, Liu Z, Sutherland JD, Sasselov DD. Origin of biological homochirality by crystallization of an RNA precursor on a magnetic surface. Sci Adv 2023;9(23):eadg8274 doi 10.1126/sciadv.adg8274.
- Ozturk SF, Bhowmick DK, Kapon Y, Sang Y, Kumar A, Paltiel Y, et al. Chirality-induced avalanche magnetization of magnetite by an RNA precursor. Nat Commun 2023;14(1):6351 doi 10.1038/s41467-023-42130-8.