Evolution
Why Dickinsonia Was Most Probably Not an Ediacaran Animal
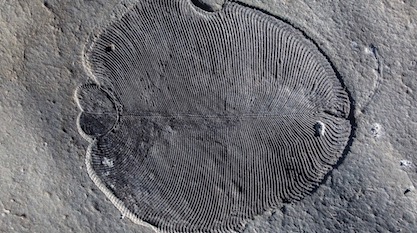
Dickinsonia is one of the iconic organisms of the strange Ediacaran biota that represent the oldest known assemblages of macroscopic organisms in the fossil record (571-541 million years ago). It has a flat, oval-shaped, bipolar, ribbed body ranging from a few millimeters up to 1.4 meters in length, exhibiting a glide reflection symmetry along an anterior-posterior axis (median ridge), maybe with differentiated dorsal and ventral side (Freeman 2009).
Although we know many well-preserved specimens of Dickinsonia, its affinities in the supposed tree of life have remained extremely controversial for more than 70 years since its description (Shu et al. 2014, Budd & Jensen 2015). This resulted in its wandering across nearly every kingdom and many phyla of life. In 1992 seven authorities among paleontologists were asked by evolutionary biologist Rudolf Raff to specify the affinities of Dickinsonia and they gave seven different answers (Raff 1996). The published alternative attributions include an independent eukaryotic kingdom Vendozoa or Vendobionta (Seilacher 1989, 1992) of quilted marine protozoans, giant protists like xenophyophore foraminiferans (Seilacher et al. 2003), terrestrial lichens or mushrooms (Retallack 1994, 2007, 2012), benthic placozoans (Rozhnov 2009, 2010, Sperling & Vinther 2010), comb jellies/ctenophores (Zhang & Reitner 2010), cnidarians (pelagic jellyfish or sessile corals or sea anemones) (Sprigg 1947, 1949, Harrington & Moore 1956, Erwin 2008, Valentine 1992, Buss & Seilacher 1994), platyhelminth/turbellarian flat worms (Termier & Termier 1968, Fedonkin 1981), annelid worms related to the recent genus Spinther (Glaessner & Wade 1966, Wade 1968, 1972, Glaessner 1979, Cloud & Glaessner 1982, Runnegar 1982, Gehling 1991, Jenkins 1992), an extinct higher animal phylum Proarticulata (Fedonkin 1985, 1990, Ivantsov 2007), or an extinct animal class Dipleurozoa (within Proarticulata) possibly related to chordates and/or nemerteans (Dzik & Ivantsov 1999, Dzik 2003, Fedonkin 2003), the sister group (together with the Ediacaran frond-like taxa) of Eumetazoa (Hoyal Cuthill & Han 2018), or a position among basal bilaterian animals (Gold et al. 2015), or an unresolved attribution to Metazoa incertae sedis (Hoekzema et al. 2017). All of these disparate attributions have been disputed by other eminent scientists.
“Holy Grail of Paleontology”
Now, a new study (Bobrovskiy et al. 2018b) claims to have finally solved this “holy grail of paleontology” (ANU TV 2018) by identifying Dickinsonia as the oldest metazoan animal and thus as the elusive prelude to the Cambrian explosion of animal body plans. This time the new hypothesis is not based on morphological comparisons but on biochemical evidence from lipid biomarkers, which is highly interesting in itself considering the estimated age of more than a half billion years for these fossils. But does the new evidence justify the bold claims made? Indeed in the past decade several papers proposed an animal nature for Dickinsonia, so let’s first briefly review this cumulative evidence before we look at the new paper. Such a review is needed because a layman could get the false impression of an emerging new scientific consensus about Dickinsonia as an Ediacaran animal.
Shared Body Symmetry with Bilaterian Animals?
All higher multicellular animals exhibit a characteristic bilateral mirror symmetry along the body axis, which is why they have been called Bilateria. From worms, to bugs, to dinosaurs and us, we all share this distinct bilateral symmetry of the right and left body sides (the five-point radial symmetry of echinoderms is a notable exception to this rule). Other organisms such as bacteria, protists, plants, fungi, sponges, placozoans, ctenophores, and cnidarians do not exhibit this bilateral symmetry.
Therefore, it was considered an interesting question whether Dickinsonia shares the bilaterian body plan with its mirror symmetry. If so, it would be a derived similarity that could be legitimately considered as evidence for a phylogenetic relationship. However, the short answer is no, yet like many a Facebook status, “It’s complicated.” Actually, paleontologists still disagree about this simple issue, though even a brief look at well-preserved fossils hardly leaves room for doubt.
Four Interpretations
The fact is that the vast majority of sufficiently well-preserved fossils of Dickinsonia clearly show a so-called glide reflection symmetry (left and right body side are not aligned but somewhat shifted along the body midline, so that there is no mirror symmetry), while only a handful of specimens seem to show bilateral symmetry with the segments apparently crossing the median body axis. This led to four very different interpretations:
- Dickinsonia had a glide symmetry, and the few apparently bilateral symmetric specimens are due to preservational artifacts;
- Dickinsonia was bilateral symmetric, and the majority of specimens with apparent glide symmetry are due to preservational artifacts;
- Dickinsonia had a rotational symmetry, with a rarely preserved ventral side with bilateral symmetry, and a more frequently preserved dorsal side with glide symmetry (Wade 1972, Gehling 1991, Jenkins 1992, Gehling et al. 2005, Brasier & Antcliffe 2008, Budd & Jensen 2017);
- Dickinsonia had a biradial symmetry like comb jellies (Zhang & Reitner 2006), which has remained an idiosyncratic fringe view.
The second hypothesis, which makes the weird claim that the very few exceptions are the true condition, clearly is not plausible. The other three hypotheses are all incompatible with a bilaterian body plan. Other Ediacaran organisms such as Yorgia, which are very similar to Dickinsonia and almost certainly closely related within the group Proarticulata, clearly show a very distinct glide symmetry beyond any reasonable doubt. This is unanimously acknowledged by all paleontologists working on Ediacaran organisms. Thus, the body symmetry of Dickinsonia definitely does not support an attribution to bilaterian animals.
Image: Different species of Dickinsonia all showing the glide symmetry, by Aleksey Nagovitsyn (User:Alnagov) [CC BY-SA 3.0 or GFDL], from Wikimedia Commons.
Glide symmetry was supported by Fedonkin (1992, 2003), Ivantsov (1999, 2007), and Brasier & Antcliffe (2008), while bilateral symmetry was suggested by Gehling et al. (2005) and Gold et al. (2015), and a rotational symmetry by Budd & Jensen (2015). But how can scientists have so different views with regard to an observed phenomenon? The answer is that there is no unbiased study of raw data, but always an interpretational perspective involved in the analysis of fossil patterns. Furthermore, the preservation is often ambiguous and leaves much room for speculation, further complicated by wishful thinking. If you want to have an Ediacaran animal ancestor, you better look for bilateral symmetry.
In Search of Bilateral Symmetry
Evans et al. (2017) claimed support for bilateral symmetry based on a few juvenile specimens featured in their Figure 2, which says in the figure legend, “Notice the clear bilateral symmetry.” The problem is that this bilateral symmetry is only clearly visible in a single one of their specimens (SAM P41202), while the others are ambiguous and either show non-aligned “ribs” or even some that seem to be confluent or branching. Furthermore, their few specimens do not rule out interpretation 3 from above.
Brasier & Antcliffe (2008) demonstrated that “slightly offset segments will appear to show ‘segment continuity’ across the axial region as the segments get smaller.” They concluded from their study of numerous specimens: “Segments can also appear to bifurcate and merge — a feature which, together with the glide plane of symmetry, is inconsistent with the bilaterian model often argued for this taxon. ” They also mention that there are two different “schools” of interpreting the Ediacaran fossils: the “Australian School,” which emphasizes the differences between Ediacaran organisms, is inclined to consider the glide symmetry as an artifact, while the “European School,” which tends to consider most of the Ediacaran organisms as closely related, is inclined to consider the glide symmetry as the correct morphology.
Gold et al. (2015) wrote:
This debate about the nature of D. costata’s metameric units has been the major challenge to those supporting a bilaterian affinity; significant disagreement still exists as to whether these units cross the midline (bilateral symmetry) or are offset (sometimes called “glide symmetry”; see Fedonkin 1984). While many smaller D. costata fossils demonstrate bilateral symmetry, larger specimens often exhibit glide symmetry. Proponents of glide symmetry suggest that offset units can generate an illusion of segment continuity in smaller individuals, particularly when organisms are preserved in coarse sediments (Brasier and Antcliffe 2008). Proponents of bilateral symmetry argue that torsion during burial and decomposition causes deformation, which produces apparent offsets of metameric units across the midline, particularly in larger individuals (Gehling et al. 2005). We personally favor the later arguments, and believe that the images presented in this article (Fig. 1) provide an unambiguous demonstration of metamere continuity across the midline, at least in smaller-sized individuals.
However, if you look at their Figure 1 it is hardly unambiguous, and to me it rather looks like glide symmetry in the specimens of Figure 1A-C, while Figure 1D indeed looks more bilateral symmetric. Even the authors acknowledge in their figure legend that the symmetry “becomes ambiguous as the size of the units approaches the grain size of the casting medium.” This is exactly the problem in all juvenile specimens, which are the only specimens claimed to show bilateral symmetry. The argument of Gehling et al. (2005) that larger specimens are all (!) deformed by torsion is clearly refuted by the perfectly preserved large specimen photographically documented by Ilya Bobrovskiy (see SBS News and below). By the way: if not due to a folding artifact, this photo also may show a unique mode of reproduction by terminal budding that has never been described for dickinsoniids in the literature and that would clearly contradict a bilaterian relationship.
Photo: Dickinsonia, by Ilya Bobrovskiy, Australian National University (fair use for scientific and educational purposes), via SBS News.
Evidence for Internal Organs?
As with the issue of symmetry, scientists are strongly divided about the internal anatomy of Dickinsonia and its Ediacaran relatives. Brasier & Antcliffe (2008: Table 1) provided a chart that showed the varying confirmation or rejection of 16 anatomical features by the authors of 10 major papers, who failed to agree on a single of these issues!
Seilacher (1989, 1992) considered Dickinsonia to share the quilted pneu construction (like an air mattress) typical for Vendobionta, together with lacking any internal organs. On the other hand, a range of paleontologists (Glaessner & Wade 1966, Glaessner 1984, Jenkins 1992, Dzik & Ivantsov 1999, 2002, Ivantsov & Malakhovskaya 2002, Dzik 2000, 2003, Ivantsov 2004) have suggested the presence of internal structures in some specimens of Dickinsonia, which they interpreted as digestive-distributive systems with metameric caeca and serial gonads. (It might be noteworthy that Jerzy Dzik is a kind of maverick fringe paleontologist notorious for very controversial interpretations.) Zhang & Reitner also described internal organs, which they considered as homologous to the meridional canals of comb jellies. Harrington & Moore (1956) considered peripheral radial markings as coelenterate tentacles.
Because the purported internal organs are “subtle features for which alternative interpretations are invariably offered” (Budd & Jensen 2007), this identification of internal organs has met with healthy skepticism from other scientists, who considered these structures as artifacts due to contractional wrinkles (Gehling et al. 2005). Gehling et al. (2005) still considered any gut, mouth, or anus to be lacking in Dickinsonia. Brasier & Antcliffe (2008) concluded, “Nor are there acceptable indications for the presence of eyes, mouth, anus, gut, parapodia, or morphologically (as opposed to taphonomically) distinct dorsal and ventral surfaces.” They clarified that “Although a groove can later be interpreted as an intestinal tract or meridional canal, reasons clearly need to be given as to why it is not interpreted as something else, for example, a plant-like vascular bundle, or a wrinkle.” They explained that “This study shows that many of the curious morphological features of Dickinsonia can be most simply explained by a hydrostatic body which maintained a high internal turgor pressure during life and which then experienced subsequent loss of turgor pressure after death.” Sperling & Vinther (2010) also emphasized that “if Dickinsonia lacks a gut and mouth … it is unlikely to be a eumetazoan.” Nevertheless, Budd & Jensen (2017) accepted the presence of internal branching body cavities, mainly because of the consistent form and symmetry of these channels, but refrained from speculating on their function. In the same year another study by Evans et al. (2017) disagreed and concluded that “there is no evidence for the number of tissue layers or the presence of a mouth, anus or any type of gut in Dickinsonia.”
Indirect Evidence
As indirect evidence for muscles, muscular contraction has been suggested by some authors (Glaessner & Wade 1966, Wade 1968, Runnegar 1982, Gehling 1991, Xiao & Laflamme 2009, Reid et al. 2018) as an explanation for strange wrinkles in some specimens. Others, though, considered the evidence unconvincing and alternatively explained by postmortal shrinkage (Brasier & Antcliffe 2008). I agree that all apparent internal structures are postmortal shrinkage artifacts of the quilted pneu “segments,” due to decay and diagenetic processes.
Based on all the disputes describe above we can safely conclude that there obviously exists no uncontroversial evidence for any specific anatomical similarity that would suggest an animal nature of Dickinsonia.
Image: Controversial internal anatomy of Dickinsonia, via Wikimedia Commons.
Did Dickinsonia Actively Move on the Sea Floor?
Supposed trace fossils of the dickinsoniids Dickinsonia and Yorgia have been described under the name Epibaion from the Ediacaran of South Australia and the Russian White Sea (Ivantsov & Malakhovskaya 2002, Gehling et al. 2005, Fedonkin 2003, Fedonkin & Vickers-Rich 2007, Sperling & Vinther 2010, Ivantsov 2011, 2013, Xiao & Knauth 2013, Evans et al. 2015). These alleged trace fossils are always represented by multiple body impressions, sometimes separated and sometimes overlapping, and often of different size and shape. These serial patterns have been interpreted as grazing traces, formed by dickinsoniids that were gliding or swimming from place to place, where they rested and fed on the microbial mat substrate by direct surface absorption through their digestive ventral sole, and thereby are thought to have produced series of distinct imprints in the microbial mats (Gehling et al. 2005, Rozhnov 2009, 2010, Sperling & Vinther 2010, Ivantsov 2011, 2013).
Dzik & Ivantsov (1999) and Dzik (2003) disagreed with this view and considered the serial touchdown imprints as made by a dead organism periodically changing its position due to slow currents before final burial under the sand. I could hardly agree more.
Retallack (2007), even though otherwise suggesting a highly controversial hypothesis himself (interpreting the traces as decayed “fairy rings” of terrestrial fungi), debunked the alleged feeding traces of Dickinsonia with these apt comments:
These are not successive displacements and reattachments or grazing traces of a single individual because they are slightly different in size (Fig. 1E). None of the supposed trails shows lateral undulation, bulldozed rims, brush marks, or backfills, that would indicate motion of a creeping or swimming organism. All these supposed trails and resting marks show ghost oval outlines, not a continuous swath of a moving individual. Lack of such indications of motion was also noted by Gehling et al. (2005), who argued for a worm-like Dickinsonia moving intermittently and feeding by means of a digestive foot, rather than a mouth. Such diffuse feeding mechanisms are characteristic of fungi, lichens and xenophyophores. These supposed trails are interpreted here as decayed specimens.
Brasier & Antcliffe (2008) concluded in their thorough redescription of Dickinsonia that “There is no evidence from within material of Dickinsonia from Ediacara, or from any other material yet known, of true escape trails, faecal trails or locomotion traces.” Likewise, McIlroy et al. (2009) concluded from their study:
This experimental study explores the preservational effects brought about by the passive movement of dead macro-organisms upon a pre-established marine microbial mat under laboratory conditions. This demonstrates the potential for making multiple impressions of body tissues on the surface of microbial mats by means of passive transport alone. This throws doubt on the evidence for active feeding in Ediacaran fossils such as Dickinsonia and Yorgia.
Sperling & Vinther (2010) disagreed and considered passive current-driven marks as unlikely, because of the non-linear pattern of the traces. Therefore, they concluded “Further, these traces readily distinguish dickinsoniids from apparently immobile Ediacaran organisms such as the rangeomorphs and erniettamorphs.” They inferred from these assumed traces a feeding mode with a ventral digestive sole, which was one of the main reasons for their attribution of Dickinsonia to the placozoan grade between sponges and bilaterian animals. However, based on a phylogenetic analysis of photo-referenced characters, Hoyal Cuthill & Han (2018) recently proposed convincing arguments for a close relationship of Dickinsonia with the sessile frond-like Ediacaran clades Rangeomorpha and Erniettamorpha, which would hardly be compatible with the placozoan hypothesis of Sperling and Vinther.
Evans et al. (2015) and Droser & Gehling (2015) considered the feeding traces as genuine and rejected the alternative explanation as random products of wave or current transport by McIlroy et al. (2009), because “repeated observation that the “footprints” were made in a definite order, as determined by identification of the body axis and anterior enlargement of body divisions in these organisms. The implication that the mat on the substrate decomposed sufficiently to enable a cast suggests that such a process could have been a source of nutrition for dickinsoniids.”
Hoekzema et al. (2017) acknowledged that the interpretation of the apparent trace fossils is an unsolved problem: “However, in the absence of direct morphological evidence for feeding mechanisms, it is not yet possible to conclude with certainty whether such traces represent feeding by ventral sole digestion as in placozoans [4], cilia-driven grazing (e.g. [29]) or even passive reclining on the surface [35].” Shockingly, this clear confession did not prevent the authors from considering the dubious trace fossils as part of a cumulative case in favor of a metazoan nature of Dickinsonia. Of course, some invalid arguments to not add up to a good argument.
The main problem with the feeding trace interpretation is very simple to summarize: there are no other fossil or living organisms known at all that leave any kind of traces that are remotely similar to the dickinsoniid pattern of distinct serial imprints. The supposed mode of origin for these traces is completely hypothetical and without any empirical support. It is nothing but a baseless fantasy imposed on enigmatic fossils.
Photo: Assumed trace of the dickinsoniid Yorgia waggoneri, via Wikimedia Commons.
Ontogenetic Growth Pattern
Hoekzema et al. (2017) published a quantitative study of the developmental biology of Dickinsonia, which allegedly confirms it as a metazoan animal, even though unlike any known today (Barras 2018). As usual, the media triumphantly proclaimed “Mystery Fossil Finally Confirmed to Be an Animal After 70 Years of Research” (Starr 2017) or “‘Mysterious’ ancient creature was definitely an animal, research confirms” (University of Oxford 2017). But do the actual results really confirm this claim? Get ready for an appalling surprise: absolutely nothing, literally nothing at all, in this new study provided any new evidence for a metazoan affinity of Dickinsonia! The authors studied the growth pattern of the 518-million-year-old Ediacaran organism Stromatoventris and suggested the new hypothesis that Dickinsonia grew from the pre-terminal deltoidal region and not via terminal addition. (Note that Evans et al. 2017, who studied 900 specimens of Dickinsonia, came to exactly the opposite conclusion, just as all previous authors including Wade 1972, Runnegar 1982, Ivantsov 2007, Retallack 2007, Sperling & Vinther 2010, Gold et al. 2015.) Hoekzema et al. did not even bother to propose any similarities with the growth patterns of metazoan animals. In their discussion they uncritically list the previously published arguments for an animal affinity and simply agree with them without adding to them whatsoever. (Actually they even admit that the apparent trace fossils are not clear evidence for locomotion.) To claim that their “quantitative study of developmental biology confirms Dickinsonia as a metazoan,” as the authors boldly did in the title of their publication, is misleading and an example of very poor scientific practice that should not have passed peer review.
Dunn et al. (2018) further elaborated on the issue of Ediacaran developmental biology. In their abstract they “conclude, based on the available evidence, that the affinities of the rangeomorphs and the dickinsoniomorphs lie within Metazoa.” In their conclusions section they even claim: “We conclude that developmental data alone allow us to identify Dickinsonia, Andiva, Yorgia and the rangeomorphs as early metazoans.” That sounds like further evidence, but in the paper you find nothing but the following poor argument:
Dickinsoniomorphs as currently defined also lack evidence of parallel modularity, and show the differentiation of new units across ontogeny, precluding algal and fungal phylogenetic affinities. When combined with trace fossil evidence for motility, and anatomical evidence (Sperling & Vinther, 2010), this developmental constraint likely requires that they are metazoan. The data of Hoekzema et al. (2017) suggest that Dickinsonia may have possessed a pre-terminal growth zone along with concurrent inflative growth in lateral units and the main growth axis, which can be reconciled with the basal and pre-terminal generative zone of extant segmented bilaterians.
That is just a negative argument that excludes algae and fungi, and the old trace fossil and anatomical arguments that have nothing to do with developmental biology, and Hoekzema’s data that can just be reconciled with a metazoan pattern. (Read as: they do not positively support a metazoan relationship, but also do not contradict it.) The latter argument also requires us to homologize the deltoidal region of Dickinsonia, that was always considered to be its anterior end, with the posterior body end of metazoan animals, for which there is not a shred of evidence. Nevertheless, developmental data alone are claimed by Dunn et al. to be sufficient to establish the metazoan relationship, and this is considering the fact that the three relevant contemporary studies (Hoekzema et al. 2017 vs Gold et al. 2015 and Evans et al. 2017) on this subject came to completely opposite results concerning the mode of growth of Dickinsonia, yet still both claimed to support a metazoan relationship.
Four Obvious Problems
Gold et al. (2015) suggested that Dickinsonia did grow by terminal addition of metameric segments and that this is a synapomorphy with bilaterian animals, largely based on the work of Jacobs et al. (2005). That is hardly surprising since David Jacobs was one of the co-authors of this study. Gold et al. also say that “if D. costata was mobile, then it appears to have moved in a direction opposite to the region of growth (Gehling et al. 2005; Freeman 2009), which is further suggestive of a posterior growth zone.” However, there are four obvious problems with their claim:
- it is dubious that metamerism belongs to the ground plan of Bilateria (to be fair, the authors address this objection);
- it is dubious if Dickinsonia was metameric at all (in the sense of segmented as proposed by Wade 1972, or only externally annulated as suggested by Sperling & Vinther 2010), and if its apparent “metamerism” is homologous to the genuine metamerism within bilaterian animals (even Hoekzema et al. 2017 explicitly admit that they do not attempt to resolve this problem);
- it is dubious that Dickinsonia did grow by terminal addition (see Hoekzema et al. 2017).
- it is dubious that Dickinsonia moved at all, so that the posterior end cannot be clearly identified by being opposite to the direction of movement.
It might be interesting to note that the interpretation of the ontogeny in Dickinsonia could be further complicated by instances of neoteny as suggested by Zakrevskaya & Ivantsov (2017).
Evans et al. (2017) suggested that “The highly regulated growth of Dickinsonia, along with features such as posterior addition, bilateral symmetry and organization around an anterior-posterior axis are characteristics found in bilaterians.” Well, any growth of a complex organism has to be highly regulated. Posterior addition conflicts with the results from Hoekzema et al. (2017) and is thus far from established. Bilateral symmetry is simply false (which everybody can easily verify by googling high-res images of Dickinsonia). And organization around an anterior-posterior axis is also found in many non-bilaterian organisms, such as plants, fungi, and even protists. Thus there is nothing to see here as well. Even Evans et al. (2017) seem to agree: “The presence of features likely characteristic of more derived bilaterians, such as large body size, and lack of those thought to be present in the ancestral bilaterian, such as any type of gut, make the placement of Dickinsonia within the stem group unlikely. However, these characters are reliant on problematic ancestral state reconstructions [49,50] so the possibility of Dickinsonia as a precursor to bilaterians cannot be ruled out.”
It is hard to believe that poor science like this passes nowadays as good science in prestigious journals. Apparently, as long as it pushes the evolutionary agenda, anything goes. If you truly care about science you should be worried even if you do not (yet) doubt Darwinian evolution. Now let’s have a closer look at the newest attempt to resolve the enigma of Dickinsonia as an animal and see if it does any better.
Image: The (now obsolete?) mainstream view of the dickinsoniid ontogeny by terminal addition, by Aleksey Nagovitsyn (Alnagov) [CC BY-SA 3.0 ], from Wikimedia Commons.
Cholesterol Biomarkers and Animal Affinity
Russian doctoral student Ilya Bobrovskiy and a research team of Professor Jochen Brocks from the Australian National University carefully collected organically preserved specimens of Dickinsonia from steep cliffs of the 558-million-year-old White Sea area of Russia. From the thin layers of organic material on these specimens the scientists could extract biomarkers that specify the type and amount of steroid lipids that were present in Dickinsonia. Contrary to the surrounding stone matrix, which mainly (70 percent) contained algal C29 sigmasteroids, they found a very high amount (85-93 percent) of C27 cholesteroids, which is considered to be a hallmark of animal cells. This is in contrast with the cells of protozoans, sponges (typically producing the C30 sterol 24-isopropylcholesterol), plants, fungi, lichens, or algae. Consequently, they identified Dickinsonia as a multicellular animal of the Placozoa + Metazoa clade and concluded that this finding “supports the idea that the Ediacaran biota may have been a precursor to the explosion of animal forms later observed in the Cambrian, about 500 million years ago.” The results were published in the journal Science (Bobrovskiy et al. 2018b) and made worldwide headlines, heralding the “earliest animals found” (ANU TV 2018, Australian National University 2018, Gramling 2018, Masterson 2018, Starr 2018, Tayag 2018). In National Geographic, Professor Brock even boldly remarked, “This was actually one of us” (Wei-Haas 2018).
This year the same research team used these methods to study the enigmatic Ediacaran fossil Beltanelliformis, and found characteristic biomarkers that suggest its origin from benthic colonial cyanobacteria (Bobrovskiy et al. 2018a). As a next project, Bobrovskiy wants to study the Ediacaran frond-like rangeomorphs. Biomarkers for steroid lipids were known for a while to be preserved as “molecular fossils” in rocks of up to 1.64 billion years of age (Gold et al. 2017, Summons & Erwin 2018), and had already been used to infer the existence of sponges from about 650 million years prior to the Cambrian explosion (Gold et al. 2016). That of course generated similar media headlines celebrating the discovery of the oldest animals (Bolton 2016, Johnson 2016). Previously, hydrocarbon biomarkers for steranes had even been reported in 2.7-billion-year-old rocks (Brocks et al. 1999), but were later rejected as contaminations (French et al. 2015), so that Brocks had to submit a correction to his paper. This author is the very same Jochen Brocks whose lab is responsible for the new claim of cholesterol in Dickinsonia. Just saying. It should also be mentioned that in the other dickinsoniid Andiva, studied by Bobrovskiy (2018b), the steroid biomarkers were confounded by background signals and harder to interpret (Summons & Erwin 2018), which may be read as: they did not give the desired result of a metazoan nature.
Incomplete Homework
Paula Welander, a microbiologist at Stanford University, who was not involved in the study, said in an interview that “The study is based on the assumption that only animals create cholesterols, which … is fair with the current data but has the potential to change as we learn more about the many forms of life on Earth” (Wei-Haas 2018). Well, apparently the scientists did not really do their homework, because we already positively know that this fundamental assumption is simply incorrect: Gonçalves Boëchat (2005) studied the biochemical composition of protists and found cholesterol to be a dominating sterol (more than 70 percent) in some bacterivore protists (e.g., Cyclidium). Kodner et al. (2008) found C27 sterols to be dominant in choanoflagellates, which are a group of protists that is considered to be most closely related to metazoan animals. Chu et al. (2008) found that protists feeding on the alga Rhodomonas salina contained 5-fold to 30-fold increased cholesterol levels. Bühring et al. (2009) mention that cholesterol is a general marker for eukaryotes and found this lipid in varying amounts in microbial mats from the Pacific Atoll Kiritimati. Weete et al. (2010) found cholesterol to be the major sterol in several unicellular fungi of the phyla Chytridiomycota and Blastocladiomycota. Finally, cholesterol even occurs in plants (Behrman & Gopalan 2005), of which some (e.g., canola) can have more than 70 percent of cholesterol in the sterol fraction of their surface. Since we do not know anything about the composition and biochemistry of the no longer existing microbial mat biota and the microbial plankton of the Ediacaran biota, we can neither exclude accumulation of cholesterol in Dickinsonia from digesting microbes or even microbial endosymbiosis (Seilacher suggested that Vendobionta depended on endosymbiotic microbes), nor a postmortem origin by taphonomic processes during fossilization.
So, do high levels of cholesterol biomarkers really suggest an animal affinity of Dickinsonia? Theoretically yes, but only if the found sterol is homologous to that of cholesterol in the bilaterian animal ground plan, and only if diagenetic artifacts (including contamination) can be excluded. Neither of which really seems to be the case.
Furthermore, there is another serious problem with Bobrovskiy et al.’s results that is emphasized by Summons & Erwin (2018):
The sterols of living organisms are produced with a single three-dimensional configuration, or stereochemistry, but the steroids preserved in sedimentary rocks are typically mixtures that include more reduced and thermodynamically stable forms, as a result of chemical transformations during burial and subsequent heating. By contrast, the Dickinsonia cholesteroids mostly have the same 5β(H) stereochemistry. The only known pathway to this steroid, informally termed coprostane, is via the steroid coprostanol, which is produced in the gut of higher mammals (10–12). Coprostane is thought to be unstable on geological time scales (13). Bobrovskiy et al. attribute the presence of these unusual steroids to reduction of Dickinsonia cholesterol by bacteria during the original decomposition of the animal. Yet, coprostanes are absent in much younger, exceptionally preserved animal fossils, where the dominant steranes are 5α(H)- cholestanes (14). The association of unusual steroids associated with Dickinsonia suggests that it may have had a distinct metabolic physiology.
In short: the found cholesteroid should neither have been preserved for more than a half billion years, nor should it be expected to be found in invertebrate animals at all, including an Ediacaran stem group representative. In my humble opinion this raises considerable doubts and rather points against a homology of the shared high cholesterol levels in Dickinsonia and metazoan animals.
Conclusions
- Is there any similarity in the body symmetry between Dickinsonia and any animals?
No! - Are there any putative homologous anatomical similarities between Dickinsonia and any animals? No!
- Is there any convincing evidence for locomotion or feeding in Dickinsonia, suggesting an animal-like behavior? No!
- Are there any homologous similarities in the inferred ontogenetic growth pattern between Dickinsonia and any animals? No.
- Is the high degree of cholesterol as a steroid biomarker a biochemical similarity between Dickinsonia and metazoan animals? Yes, but it is not conclusive and there is evidence against a homology of this similarity.
- Is there any similarity between Dickinsonia and the frond-like Ediacaran organisms, which clearly have a quilted structure, fractal growth, glide symmetry, and a sessile lifestyle with holdfast in the sea floor? Yes, Dickinsonia shares the quilted structure and glide symmetry, but not the fractal growth that seems to be restricted to the frond-like rangeomorphs (Hoyal Cuthill & Conway Morris 2014).
After over seventy years of research on hundreds of well-preserved specimens, we still do not know with any degree of certainty if Dickinsonia has a distinct front and back end like animals, if it had differentiated dorsal and ventral sides like animals, if it was bilateral symmetric or not, if it had internal organs or not, if it could move or not, if it did actively feed or not, from which side it did grow, and how it reproduced. The very existence of two different “schools” of interpretation of Ediacaran organisms (see above) indicates that much of this interpretation is heavily biased by preconceptions, being prone to see in the fossils what is expected to be there. Now we can add to all this mess of uncertainty that Dickinsonia may have shared a lipid with (certainly unrelated) mammals, and moreover a lipid that should not have been preserved in the first place.
Altogether, there is hardly convincing evidence that Dickinsonia was an animal, but substantial evidence that it belonged to an “alien” clade of Ediacaran organisms with a distinct body plan and without a clear relationship to later forms of life. As Daley et al. (2018) recently confirmed, “BSTs from the latest Ediacaran Period (e.g., Miaohe biota, 550 Ma) are abundantly fossiliferous with algae but completely lack animals, which are also missing from other Ediacaran windows, such as phosphate deposits (e.g., Doushantuo, 560 Ma).” As we have seen before, the vastly exaggerated headlines in the popular science press, celebrating the revelation of Dickinsonia as the oldest animal, were at least a bit premature.
Paleontologist Mary Droser from the University of California claimed in a press comment on the new study that “Coupled with everything else, it is really hard to argue that this wasn’t an animal” (Wei-Haas 2018). Well, as I hope you have learned from this lengthy article, this is not really true at all. But even if Dickinsonia and other Ediacaran organisms were indeed to be confirmed as Metazoa with a phylogenetic position somewhere between sponges and bilaterian animals (as suggested by Evans et al. 2017), it would do nothing to explain the sudden appearance of the animal body plans during the Cambrian explosion. Ediacaran organisms, whatever they were, definitely were not transitional to any of the Cambrian animal phyla. At best they could represent a weird extinct dead-end side branch of placozoans and/or coelenterates.
In forthcoming articles here I hope to show that all the other alleged evidences for Ediacaran animals (e.g., Kimberella and vermiform trace fossils) do not stand up to scrutiny.
References:
- ANU TV 2018. Is Dickinsonia our oldest ancestor?
- Australian National University 2018. Fat from 558 million years ago reveals earliest known animal. Phys.org September 20, 2018.
- Barras C 2018. These half-billion-year-old creatures were animals — but unlike any known today. Science Aug. 8, 2018.
- Behrman EJ, Gopalan V 2005. Cholesterol and Plants. Journal of Chemical Education 82(12), 1791–1793, doi: 10.1021/ed082p1791.
- Bobrovskiy I, Hope JM, Ivantsov A, Nettersheim BJ, Hallmann C, Brocks JJ 2018b. Ancient steroids establish the Ediacaran fossil Dickinsonia as one of the earliest animals. Science 361, 1246–1249, doi: 10.1126/science.aat7228 (with Supplementary Materials).
- Brasier M, Antcliffe J 2008. Dickinsonia from Ediacara: a new look at morphology and body construction. Palaeogeography, Palaeoclimatology, Palaeoecology 270, 311–323, doi:10.1016/j.palaeo.2008.07.018.
- Bolton D 2016. Sea sponges were the first animals on Earth, scientists discover. The Independent 23 February 2016.
- Brocks JJ, Logan GA, Buick R, Summons RE 1999. Archean molecular fossils and the early rise of eukaryotes. Science 285, 1033–1036, doi: 10.1126/science.285.5430.1033.
- Budd GE, Jensen S 2015. The origin of the animals and a ‘Savannah’ hypothesis for early bilaterian evolution. Biological Reviews 92, 446–473, doi: 10.1111/brv.12239.
- Bühring SI, Smittenberg RH, Sachse D, Lipp JS, Golubic S, Sachs JP, Hinrichs K-U, Summons RE 2009. A hypersaline microbial mat from the Pacific Atoll Kiritimati: insights into composition and carbon fixation using biomarker analyses and a 13C‐labeling approach. Geobiology 7(3), 308-323, doi: 10.1111/j.1472-4669.2009.00198.x.
- Buss LW, Seilacher A 1994. The Phylum Vendobionta: a sister group of the Eumetazoa? Paleobiology 20(1), 1–4, doi: 10.1017/S0094837300011088.
- Chu FLE, Lund ED, Littreal PR, Ruck KE, Harvey E, Le Coz JR, Marty Y, Moal J 2008. Sterol production and phytosterol bioconversion in two species of heterotrophic protists, Oxyrrhis marina and Gyrodinium dominans. Marine Biology 156, 155–169, doi: 10.1007/s00227-008-1072-2.
- Cloud P, Glaessner MF 1982. The Ediacarian period and system: Metazoa inherit the Earth. Science 217, 783–792, doi: 10.1126/science.217.4562.783.
- Daley AC, Antcliffe JB, Drage HB, Pates S 2018. Early fossil record of Euarthropoda and the Cambrian Explosion. PNAS, 9 pp., doi: 10.1073/pnas.1719962115.
- Droser ML, Gehling JG 2015. The advent of animals: The view from the Ediacaran. PNAS 112(16), 4865-4870, doi: 10.1073/pnas.1403669112.
- Dunn FS, Liu AG, Donoghue PCJ 2018. Ediacaran developmental biology. Biological Reviews 93, 914–932, doi: 10.1111/brv.12379.
- Dzik J 2000. The origin of the mineral skeleton in chordates. Evolutionary Biology 31, 105–154, doi: 10.1007/978-1-4615-4185-1_3.
- Dzik J 2003. Anatomical information content in the Ediacaran fossils and their possible zoological affinities. Integrative and Comparative Biology 43, 114–126, doi: 10.1093/icb/43.1.114.
- Dzik J, Ivantsov AY 1999. An asymmetric segmented organism from the Vendian of Russia and the status of the Dipleurozoa. Historical Biology 13(4), 255–168, doi: 10.1080/08912969909386585.
- Dzik J, Ivantsov AY 2002. Internal anatomy of a new Precambrian dickinsoniid dipleurozoan from northern Russia. Neues Jahrbuch für Geologie und Paläontologie Monatshefte 2002, 385–396. (PDF)
- Erwin DH 2008. Wonderful Ediacarans, wonderful cnidarians? Evolution & Development 10(3), 263–264, doi: 10.1111/j.1525-142X.2008.00234.x.
- Evans SD, Droser ML, Gehling JG 2015. Dickinsonia liftoff: evidence of current derived morphologies. Palaeogeography, Palaeoclimatology, Palaeoecology 434, 28–33, doi: 10.1016/j.palaeo.2015.02.006.
- Evans SD, Droser ML, Gehling JG 2017. Highly regulated growth and development of the Ediacara macrofossil Dickinsonia costata. PLoS One 12, e0176874, doi: 10.1371/journal.pone.0176874.
- Fedonkin MA 1981. Belomorskaya biota Venda. Trudy Akademii Nauk SSSR 342, 1–100.
- Fedonkin MA 1985. Systematic description of Vendian Metazoa. pp. 70–106 in: Sokolov BS, Iwanowski AB (eds). Vendian System: Historical–Geological and Paleontological Foundation, Vol. 1: Paleontology. Nauka, Moscow [in Russian].
- Fedonkin MA 1990. Systematic description of the Vendian Metazoa. pp. 71–120 in: Sokolov B, Ivanovski A (eds.). The Vendian System 1, Paleontology. Springer, New York.
- Fedonkin MA 1992. Vendian faunas and the early evolution of Metazoa. pp. 87–129 in: Lipps JH, Signor PW (eds). Origin and Early Evolution of the Metazoa. Plenum Press, New York.
- Fedonkin MA (2003). The origin of Metazoa in the light of the Proterozoic fossil record. Paleontological Research 7(1), 9–41, doi: 10.2517/prpsj.7.9.
- Fedonkin MA, Vickers-Rich P 2007. First trace of motion. pp. 205–216 in: Fedonkin MA, Gehling JG, Grey K, Narbonne GM, Vickers-Rich P (eds.). The Rise of Animals. Johns Hopkins University Press, Baltimore.
- Freeman G 2009. The rise of bilaterians. Historical Biology 21(1-2), 99–114, doi: 10.1080/08912960903295843.
- French KL et al. 2015. Reappraisal of hydrocarbon biomarkers in Archean rocks. PNAS 112, 5915–5920, doi: 10.1073/pnas.1419563112.
- Gehling JG 1991. The case for the Ediacaran fossil roots to the Metazoan tree. Memoirs Geological Society of India 20, 181–224. (PDF)
- Gehling JG, Droser ML, Jensen SR, Runnegar B 2005. Ediacara organisms: relating form to function. pp. 43–66 in: Briggs DEG (ed). Evolving Form and Function: Fossils and Development: Proceedings of a symposium honoring Adolf Seilacher for his contributions to paleontology, in celebration of his 80th birthday. Yale University Press, New Haven, 288 pp.
- Glaessner MF 1979. Biogeography and Biostratigraphy: Precambrian. pp. 79–118 in: Robinson RA, Teichert C (eds), Treatise on Invertebrate Paleontology: Part A: Fossilization (Taphonomy), Biogeography and Biostratigraphy. University of Kansas and Geological Society of America, Colorado.
- Glaessner MF 1984. The Dawn of Animal Life: a Biohistorical Study. Cambridge University Press, Cambridge.
- Glaessner MF, Wade M 1966. The Late Precambrian fossils from Ediacara, South Australia. Palaeontology 9, 599–628. (PDF)
- Gold DA, Runnegar B, Gehling JG, Jacobs DK (2015). Ancestral state reconstruction of ontogeny supports a bilaterian affinity for Dickinsonia. Evolution & Development 17, 315–324, doi: 10.1111/ede.12168.
- Gold DA, Grabenstatter J, Mendoza Ad, Riesgo A, Ruiz-Trillo I, Summons RE 2016. Sterol and genomic analyses validate the sponge biomarker hypothesis. PNAS 113(10), 2684–2689, doi: 10.1073/pnas.1512614113.
- Gold DA, Caron A, Fournier GP, Summons RE 2017. Paleoproterozoic sterol biosynthesis and the rise of oxygen. Nature 543, 420–423, doi:10.1038/nature21412.
- Gonçalves Boëchat I 2005. Biochemical Composition of Protists: Dependency on Diet and Trophic Mode and Consequences for their Nutritional Quality. Dissertation Humboldt-University Berlin. (PDF)
- Gramling C 2018. Cholesterol traces suggest these mysterious fossils were animals, not fungi. Science News September 20, 2018.
- Harrington HJ, Moore RC 1956. Medusa of the Hydroidea. pp. 77–80 in: Moore RC (ed). Treatise on Invertebrate Paleontology, Part F: Coelenterata. GSA and University of Kansas Press, Kansas.
- Hoekzema RS, Brasier MD, Dunn FS, Liu AG 2017. Quantitative study of developmental biology confirms Dickinsonia as a metazoan. Proceedings of the Royal Society B 284, 20171348, doi: 10.1098/rspb.2017.1348.
- Hoyal Cuthill JF, Conway Morris S 2014. Fractal branching organizations of Ediacaran rangeomorph fronds reveal a lost Proterozoic body plan. PNAS 111(36), 13122–13126, doi: 10.1073/pnas.1408542111.
- Hoyal Cuthill JF, Han J 2018. Cambrian petalonamid Stromatoveris phylogenetically links Ediacaran biota to later animals. Palaeontology 2018, 1–11, doi: 10.1111/pala.12393.
- Ivantsov AY 1999. A New Dickinsonid from the Upper Vendian of the White Sea Winter Coast (Russia, Arkhangelsk Region). Paleontological Journal 33(3), 211–221. (PDF)
- Ivantsov AY 2004. New Proarticulata from the Vendian of Arkhangelsk Region. Paleontological Journal 38(3), 247–253. (PDF)
- Ivantsov AY 2007. Small Vendian transversely Articulated fossils. Paleontological Journal 41(2), 113–122, doi: 10.1134/S0031030107020013.
- Ivantsov AY 2011. Feeding Traces of Proarticulata – the Vendian metazoa. Paleontological Journal 45(3), 237–248.
- Ivantsov AY 2013. Trace Fossils of Precambrian Metazoans “Vendobionta” and “Mollusks”. Stratigraphy and Geological Correlation 21(3), 252–264, doi: 10.1134/S0869593813030039.
- Ivantsov AY, Malakhovskaya YE 2002. Giant Traces of Vendian Animals. Doklady Earth Sciences 385(6), 618–622. (PDF)
- Jacobs DK., Hughes NC, Fitz-Gibbon ST, Winchell CJ 2005. Terminal addition, the Cambrian radiation and the Phanerozoic evolution of bilaterian form. Evolution & Development 7, 498–514, doi: 10.1111/j.1525-142X.2005.05055.x.
- Jenkins RJF 1992. Functional and ecological aspects of Ediacaran assemblages. pp. 131–176 in: Lipps JH, Signor PW (eds). Origin and Early Evolution of the Metazoa. Plenum Press, New York.
- Johnson SK 2016. Sponge full of cholesterol pushes animal life back to 650 million years. Ars Technica 2/26/2016.
- Kodner RB, Summons RE, Pearson A, King N, Knoll AH 2008. Sterols in a unicellular relative of the metazoans. PNAS 105(29), 9897–9902, doi: 10.1073/pnas.0803975105.
- Li G 2018. Biomarker evidence that Dickinsonia were first animals. Chemistry World September 21, 2018.
- Masterson A 2018. Is this the oldest animal on Earth? Cosmos Magazine 21 September 2018.
- McIlroy D, Brasier MD, Lang AS 2009. Smothering of microbial mats by macrobiota: implications for the Ediacara biota. Journal of the Geological Society 166, 1117–1121, doi: 10.1144/0016-76492009-073.
- Raff R 1996. The Shape of Life. Genes, Development, and the Evolution of Animal Form. The University of Chicago Press, Chicago, p. 520.
- Reid LM, García-Bellido DC, Gehling JG 2018. An Ediacaran opportunist? Characteristics of a juvenile Dickinsonia costata population from Crisp Gorge, South Australia. Journal of Paleontology 92(3), 313–322, doi: 10.1017/jpa.2017.142.
- Retallack GJ 1994. Were the Ediacaran fossils lichens? Paleobiology 20(4), 523–544, doi: 10.1017/S0094837300012975.
- Retallack GJ 2007. Growth, decay and burial compaction of Dickinsonia, an iconic Ediacaran fossil. Alcheringa 31, 215–240, doi: 10.1080/03115510701484705.
- Retallack GJ 2012. Ediacaran life on land. Nature 493, 89–92, doi: 10.1038/nature11777.
- Rozhnov SV 2010. From Vendian to Cambrian: the beginning of morphological disparity of modern metazoan phyla. Russian Journal of Developmental Biology 41, 357–368, doi: 10.1134/S1062360410060032.
- Rozhnov SV 2009. Development of the trophic structure of Vendian and early Paleozoic marine communities. Paleontological Journal 43, 1364–1377, doi: 10.1134/S0031030109110021.
- Runnegar B 1982. Oxygen requirements, biology and phylogenetic significance of the late Precambrian worm Dickinsonia, and the evolution of the burrowing habit. Alcheringa 6: 223–239, doi: 10.1080/03115518208565415.
- Seilacher A 1989. Vendozoa: Organismic construction in the Proterozoic biosphere. Lethaia 22(3), 229–239, doi: 10.1111/j.1502-3931.1989.tb01332.x.
- Seilacher A 1992. Vendobionta and Psammocorallia: Lost constructions of Precambrian evolution. Journal of the Geological Society 149, 607–613, doi: 10.1144/gsjgs.149.4.0607.
- Seilacher A, Grazhdankin D, Legouta A 2003. Ediacaran biota: The dawn of animal life in the shadow of giant protists. Paleontological Research 7(1), 43–54, doi: 10.2517/prpsj.7.43.
- Shu D, Isozaki Y, Zhang X, Han J, Maroyama S 2014. Birth and early evolution of metazoans. Gondwana Research 25, 884–895, doi: 10.1016/j.gr.2013.09.001.
- Sperling EA, Vinther J 2010. A placozoan affinity for Dickinsonia and the evolution of late Proterozoic metazoan feeding modes. Evolution & Development 12, 201–209, doi: 10.1111/j.1525-142X.2010.00404.x.
- Sprigg RC 1947. Early Cambrian (?) Jellyfishes from the Flinders Ranges, South Australia. Transactions of The Royal Society of South Australia 71(2), 212–224.
- Sprigg RC 1949. Early Cambrian “Jellyfishes” of Ediacara, South Australia and Mouth John, Kimberley District, Western Australia. Transactions of The Royal Society of South Australia 73(1), 72–99.
- Starr M 2017. Mystery Fossil Finally Confirmed to Be an Animal After 70 Years of Research. Science Alert 18 Sep 2017.
- Starr M 2018. This Eerie Creature Was Just Officially Confirmed as The World’s Earliest Known Animal. Science Alert 20 Sep 2018.
- Summons RE, Erwin DH 2018. Chemical clues to the earliest animal fossils. Science 361, 1198–1199, doi: 10.1126/science.aau9710.
- Tayag Y 2018. New Timeline for Earliest Known Animal Is ‘Holy Grail’ of Paleontology. Inverse September 20, 2018.
- Termier H, Termier G 1968. Evolution et biocinèse: les invertébrés dans l’histoire du monde vivant. Masson, Paris. pp. 62–67.
- University of Oxford 2017. ‘Mysterious’ ancient creature was definitely an animal, research confirms. Press release 14 Sep 2017.
- Valentine JW 1992. Dickinsonia as a polypoid organism. Paleobiology 18: 378–382, doi: 10.1017/S0094837300010952.
- Wade M 1968. Preservation of soft-bodied animals in Precambrian sandstones at Ediacara, South Australia. Lethaia 1, 238–267, doi: 10.1111/j.1502-3931.1968.tb01740.x.
- Wade M 1972. Dickinsonia: polychaete worms from the late Precambrian Ediacara fauna, South Australia. Memoirs of the Queensland Museum 16(2): 171–190.
- Weete JD, Abril M, Blackwell M 2010. Phylogenetic distribution of fungal sterols. PLoS One 5(5), e10899, doi: 10.1371/journal.pone.0010899.
- Wei-Hass M 2018. This Enigmatic Blob Was One of Earth’s Earliest Animals. National Geographic September 20, 2018.
- Xiao S, Knauth LP 2013. Palaeontology: fossils come in to land. Nature 493, 28–29, doi: 10.1038/nature11765.
- Xiao S, Laflamme M 2009. On the eve of animal radiation: phylogeny, ecology and evolution of the Ediacara biota. Trends in Ecology & Evolution 24(1), 31–40, doi: 10.1016/j.tree.2008.07.015.
- Zakrevskaya M, Ivantsov AY 2017. Dickinsonia costata — the first evidence of neoteny in Ediacaran organisms. Invertebrate Zoology 14(1), 92–98, doi: 10.15298/invertzool.14.1.13.
- Zhang X, Reitner J 2010. A fresh look at Dickinsonia: removing it from Vendobionta. Acta Geologica Sinica 80, 636–642, doi: 10.1111/j.1755-6724.2006.tb00286.x.
Photo at top: Dickinsonia costata from the Ediacaran of Australia, by Verisimilus at English Wikipedia [GFDL, CC-BY-SA-3.0 or CC BY 2.5 ], from Wikimedia Commons.