Intelligent Design
Life Sciences
Cellulose Doesn’t Just Happen
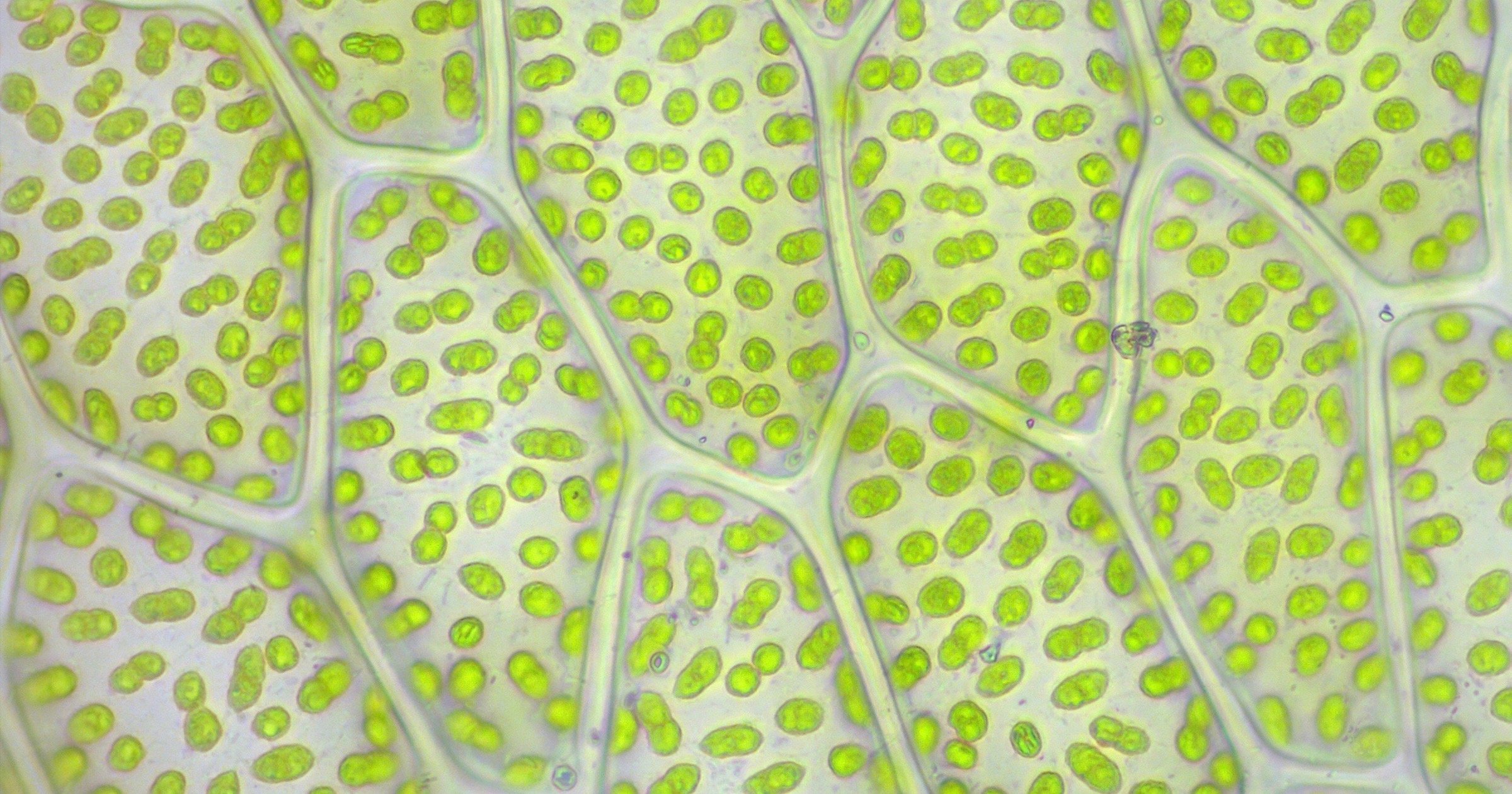
“Wood” you believe that the most abundant biopolymer on Earth requires a host of machines, genes, proteins, and accessories? Cellulose is only made by life. It doesn’t emerge fully formed from volcanoes or abiotic chemistry. To paraphrase Aristotle, if the art of cellulose manufacture were within atoms, we would have cellulose by the nature of atomic physics.
(Aristotle was reasoning that something else than raw nature was needed for shipbuilding: namely, art, which presupposes intelligence and purpose. “If the art of ship-building were in the wood,” he quipped, “we would have ships by nature.”)
Cellulose is most commonly understood as the chief component of the cell walls of plants. It is also manufactured, however, by some microbes like bacteria and algae, fungi and slime molds, and urochordates (tunicates). Each organism makes cellulose according to its needs; bacteria, for example, do not need the extra machinery to make long fibrils that plants need.
In a Primer in Current Biology, Lise C. Noack and Staffan Persson (hence N&P) described “Cellulose synthesis across kingdoms.” As evolutionists, they attribute the art of cellulose manufacture to evolution: “Other proteins evolved before the emergence of the hexameric rosette structure,” they say in one place.
Cellulose synthesis is present in all kingdoms of life and is characterized by an evolutionarily conserved BcsA/CesA synthase. [Emphasis added.]
Evolutionary conservation is not evolutionary at all, it goes without saying; it means stasis. But having asserted that cellulose synthesis emerged and evolved (or not), the hard work of explaining its origin is put off the table. Most of the article deals with how cellulose is made.
Building Blocks on Other Building Blocks
The basic building block of cellulose is the sugar glucose, a six-carbon ring structure with the formula C6H12O6. Notably, glucose is not found in abiotic nature either. It is only a product of living cells. Although NASA has claimed some sugars have been detected in meteorites, I could find no reference to glucose being formed outside of living organisms.
One NIH paper from 2022 starts, “Gluconeogenesis is the pathway by which glucose is formed from non-hexose precursors such as glycerol, lactate, pyruvate, and glucogenic amino acids.” Already we see, even before cellulose synthesis begins, its monomer glucose must be “formed” by a “pathway” in a living cell. Those words suggest an organized process that assembles prior building blocks. N&P bypass that point, assuming the prior existence of glucose in the cell:
Cellulose consists of glucose molecules connected through beta-1,4-acetal linkages, which are generated by cellulose synthases and result in the formation of unbranched glucan chains.
Bacterial Cellulose Synthase
Surprisingly, N&P’s Figure 1 shows more components in the bacterial synthase machinery than in the plant machinery.
The protein complex that synthesizes cellulose was first discovered in bacteria, where it consists of a core complex composed of two subunits — BcsA and BcsB — and many accessory proteins, the presence of which varies depending on bacterial species (Figure 1A). BcsA is strictly speaking the cellulose synthase because it carries the cytosolic glycosyltransferase domain, as well as a transmembrane domain that allows for cellulose translocation and a regulatory carboxy-terminal PilZ domain that senses cyclic di-GMP (Figure 2A).
We’re just getting started, and already a supply of previously manufactured glucose molecules are needed in the right place at the right time, where the machinery is embedded in the bacterial inner membrane. Then we need the protein complex BcsA with its two subunits, and “many accessory proteins.” But getting the parts list right is only a beginning. The parts have to work together in functional harmony.
The machinery needs to link the glucose molecules together and then translocate them to the outer membrane. This is done by two more protein complexes, BcsB and BcsC. They won’t work correctly without another component: a regulator that senses cyclic di-GMP, abbreviated c-di-GMP. N&P gloss over that detail, so now we must look that up. Nature Reviews says,
c-di-GMP controls cellular processes at the transcriptional, translational and post-translational level, and through an increasing number of c-di-GMP-binding proteins and riboswitches.
We have lost count of the number of components to make cellulose and get it moved to where it is needed, and this is in a bacterium! Consider just one of the other machines:
BcsB is the cocatalytic subunit or co-polymerase because its presence is required for cellulose polymerization. It contains a periplasmic carbohydrate-binding domain that might guide the glycan chain towards the outer membrane secretory components.
The term cocatalytic implies cooperation between machines. This component, furthermore, must guide the chain to where it is needed. Another machine, BcsZ, regulates the arrangement of the polymers.
Plant Cellulose Synthase
The cellulose machinery in plants has fewer components but more functional requirements. It doesn’t need the two translocators BcsB and BcsC, because the polymers go directly from the inner membrane to the cell wall. Instead of the polymerase BcsA, plants and some algae combine the glucose molecules into polymers with a machine called CesA.
The authors speculate about a possible ancestral relationship between CesA and the bacterial BcsA synthase, but admit that “the phylogenetic relevance of terminal complex organization is still somewhat unclear.” Whatever; CesA in plants is arranged in geometrically-perfect “rosettes” of six sets of 3 CesA domains held together with three other proteins, PCR, CSR, and NTD. The rosette structure gives plant cellulose its cable-like formation, woven like strands of a rope. These cables confer the strength needed to support tall trees.
At the risk of getting too deep in the weeds, this quote gives a taste of the complexity of making cellulose. Conserved, remember, means not evolved.
At the level of the amino-acid sequence, the glycosyltransferase domain has four conserved regions: the first three each contain a D residue, whereas the fourth contains a Q(Q/R)xRW motif. The resulting D–D–D–Q(Q/R)xRW motif is conserved in all BcsA and CesA proteins and is essential for glycosyltransferase function. This highlights a remarkable conservation from sequence to tertiary protein folding, indicative of a conserved enzymatic mechanism. Apart from the D–D–D–Q(Q/R)xRW motif, plant and some algae CesA proteins have three additional domains that are likely involved in protein oligomerization: an amino-terminal RING-like zinc-finger domain (NTD), a plant-conserved region (PCR) and a class-specific region (CSR) (Figure 2B). Although the role of the NTD in CesA oligomerization is still unclear, the PCR and CSR domains are thought to be responsible for the rosette architecture of the cellulose synthase complex in plants (Figure 2C).
N&P discuss some of the similarities and differences in these machines within different species. Some parts are interchangeable, they note. Those details do not affect the overall impression that many parts are needed to make cellulose. Bacterial cellulose polymers can be less organized, because they contribute to biofilm formation. In plants, though, the polymers are shaped into microfibrils, sheets, or ribbons.
There is a strong correlation between terminal complex organization and microfibril dimensions. Rosette CesA complexes from plants and algae form small-diameter microfibrils ranging from 2 to 3.5 nm. However, single or multiple row arrangements of terminal complexes can give rise to much wider and thicker microfibrils, up to 25 nm in diameter, or flat ribbons of cellulose up to 100 nm in width. Depending on the structure, cellulose microfibrils engage with a variety of other polysaccharides and glycoproteins to form complex networks.
Just when our heads are spinning trying to remember all the parts, N&P discuss “Additional subunits of the cellulose synthase complexes.” I count at least 17 more proteins “involved in different regulatory aspects of cellulose synthesis.”
Let’s recap the importance of cellulose with this quote from a chemistry lesson from Imperial College London:
Cellulose is another glucose polymer (molecular weight 150,000-1 million) found in the cell walls of plants. Over 50% of the total organic matter in the world is cellulose. For example, wood is about 50% cellulose, and cotton is almost 100% cellulose. It is a strong, rigid linear molecule, and these features allow it to be used as the main structural support for plants. The glucose units are again held together by linkages, but this time every second glucose unit is flipped over. These links are called b,1:4 linkages, and human bodies do not possess the enzymes necessary to break this bond. Therefore any cellulose we eat passes through the digestive tract undigested, and acts as roughage. Grass feeding animals, such as cows, however, can digest cellulose, since they have extra stomachs to contain the grass for long periods while it is broken down by special bacteria.
Because of the enormous number of parts, machines and regulators involved in cellulose manufacture, we have wood, lumber, and shipbuilding. The art of shipbuilding may not be in the wood, but what would Aristotle have thought about the art of cellulose manufacture therein?