Evolution
Intelligent Design
Physics and Chemistry Could Not Give Rise to Biology
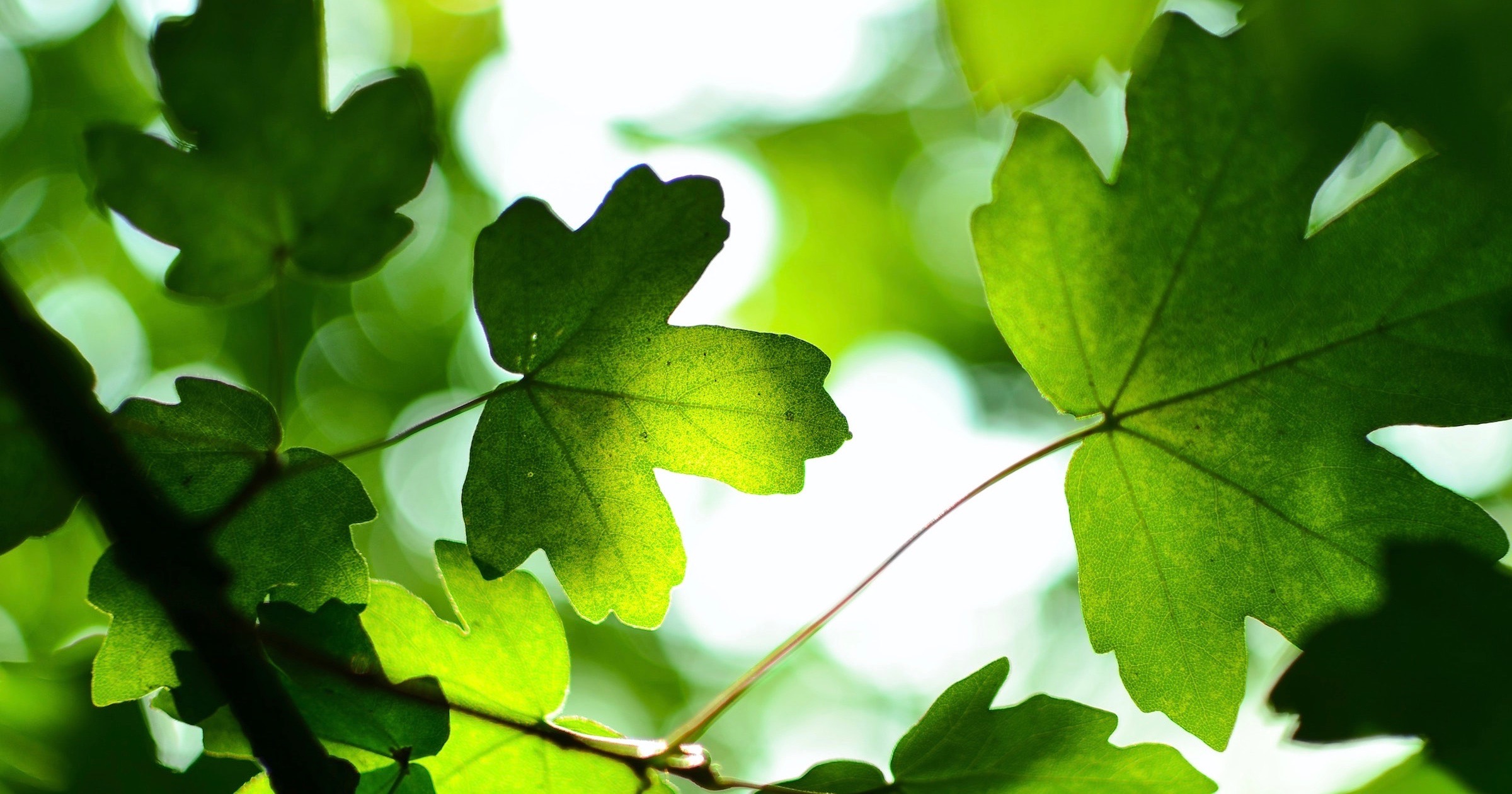
The laws of nature provide stable conditions and physical boundaries within which biological outcomes are possible. Laws are, in effect, a chessboard. They provide a stable platform and non-negotiable boundaries. But they do not determine the movement of pieces or the outcome of the game.
Or do they? Rope Kojonen, a theologian at the University of Helsinki, argues for the compatibility of design and evolution. My colleagues Steve Dilley, Brian Miller, Casey Luskin, and I published a review of Kojonen’s thoughtful book, The Compatibility of Evolution and Design, in the journal Religions. In a series at Evolution News, we have been expanding on our response to Dr. Kojonen. Here, I will shift gears to analyze his claims about the laws of nature and their role in the origin of biological complexity and diversity.
Mechanisms of Design
The laws of nature are at the heart of Kojonen’s model. They are the mechanisms of design, the linchpin of Kojonen’s project to wed design and evolution. To evaluate his model, however, we need to be clear about what exactly his position is. Kojonen is not entirely clear about how the laws of nature (and initial conditions) are said to bring about the origin of life, the diversification of life, and human cognition. However, there seem to be at least three possible ways to interpret Kojonen’s model:
- The laws of nature gave rise to “laws of forms” and other preconditions, which allowed selection and mutation (along with other processes) to create all biological complexity and diversity, including nucleotide sequences, new proteins, the assembly of protein machines, intricately engineered motility, and navigation systems, and all the unique capabilities possessed by humans. In this view, the laws of nature (in the main) have causal power or limit the possibility space enough that organisms emerge. Notably, this process isn’t deterministic per se. On this interpretation, there is room for environmental conditions to work alongside laws of nature to shape what evolutionary pathways are available, what kind of structures are easier to evolve, and so on. Insights from structuralism, convergence, and evolutionary algorithms apparently provide details about how this might work. But, the bottom line with this interpretation is that the laws of nature and environmental conditions play a generative role in bringing about flora and fauna. The laws of nature, environmental conditions, and so on don’t simply transmit biologically relevant “information” built into the initial conditions of the Big Bang. Instead, they actually create more biologically relevant information.
- The laws of nature simply transmitted biologically relevant information sufficient to produce all biological complexity and diversity, including new proteins, protein machines, and the like. This biologically relevant information was “built in” to the mass-energy configuration at the big bang. The laws of nature did not create anything but rather were the media (or “carriers”) through which biologically relevant information was eventually expressed and instantiated in everything from proteins to bacterial flagella to human beings.
- The laws of nature gave rise to “platonic” forms, which then constrained evolution in ways that allowed selection and mutation to build biological forms. These “forms” are “an emergent consequence of the laws of chemistry and physics.” In this interpretation, the laws created these forms. The forms themselves are more than simply laws and matter under a different guise; they are non-physical. In this view, laws generated these forms, which then shaped the physical tendencies of matter such that (with possible contingency and other factors in play) they produced biological information sufficient for selection and mutation to evolve all manner of proteins, protein machines, unique human abilities, and the like.
Let’s discuss point one, namely, that the laws of nature (and the like) have causal power or limit the possibility space enough that the diversity of plant and animal species observed today emerged from unicellular organisms. While I am personally convinced that design is evident in the very fabric of the universe and yes, in the laws of physics and chemistry, these material mechanisms do not have sufficient causal power or limit the possibilities sufficiently to explain how the diversity of organisms came to be (if these laws have stayed the same over time). To support this point, I’ll talk about the capabilities of the laws of physics and chemistry and give examples of how they currently interact with biology.
In Kojonen’s model, the laws of nature do the heavy lifting in terms of creating biological complexity. While Kojonen cites an array of other factors — e.g., environmental conditions, structuralism, convergence, and evolutionary algorithms — it’s also clear that these factors are undergirded by the laws of nature themselves. But there are limits to the creative power of the laws of nature. If it turns out that the laws have limited ability to produce biological complexity, then other factors (such as the environment, convergence, etc.) that depend upon the laws of nature likewise have limits. If Kojonen thinks that these other factors have creative powers that transcend the limits of the laws of nature, then the burden is on him to show that. Is it possible for the laws of nature to be a causal force or sufficiently constrain the possibility space?
Material Mechanisms
According to one definition, a mechanism is a process that acts on objects to produce an outcome. Here I will define a material mechanism as a process by which a physical object is acted upon by one of the physical laws. Material objects are built from the elements of the periodic table, and the laws of physics and chemistry are the constant processes that constrain how material objects behave. To understand materialistic mechanisms, let’s look at a few illustrations.
The Law of Gravity
Definition: The law of universal gravitation says an object will attract another object proportionally to the product of their masses and inversely related to the square of their distance from each other.
This law tells us how objects behave toward one another. Gravity constrains motion, whether that motion is human, planetary, or light. A complex system may also be able to detect gravity and use it as a cue. Let’s look at an example of plant growth. Leaves grow in the opposite direction of gravitational pull, but roots grow downward in the direction of gravitational pull. What causes this? Is it gravity? Definitely not. Root growth occurs through the division of stem cells in the root meristem, located at the tip of the root. Thus, root stem cells rely on gravity as a cue to be detected by their sensors, so that they know where to direct their growth. But gravity is not the mechanism that creates plant morphology. Rather, plants work within the constraints of gravity and exploit it via sensors to scaffold their architecture.
Electrostatic Laws
Definition: The electrostatic laws state that charges attract or repel with a force that is proportional to the product of their charges and inversely proportional to the square of the distance between them, depending on whether they are alike or different.
Electrostatic laws describe the attraction of positively charged ions to negatively charged ions. These laws constrain (but do not cause) the way an electrochemical gradient can be formed and work across a membrane. The charge and concentration differential across a membrane creates an electrical field. The cell then uses the potential energy of the electrical field to generate energy, convey electrical signals, and power the delivery of nutrients into the cell. The crucial point here is that electrochemical gradients are not an emergent property of the electrostatic laws. Instead, they are caused by molecular machinery. As Elbert Branscomb and Michael J. Russell say in a recent BioEssays paper, “to function, life has to take its transformations out of the hands of chemistry and operate them itself, using macromolecular “mechano-chemical” machines, requiring one machine (roughly) for each transformation; life must, in Nick Lane’s evocative phrasing, “transcend chemistry.” (Branscomb and Russell 2018)
How do electrostatic laws interface with organisms’ body plans? Organism body patterning is formed in part by bioelectrical networks, which operate across cell fields to integrate information and mediate morphological decision-making. (Djamgoz and Levin 2022) The bioelectrical networks play critical roles by regulating gene expression, organ morphogenesis, and organ patterning. This is, of course, exactly what would be necessary as an emergent property from electrostatic laws for them to have generative capacity. But these bioelectric networks no more emerge from the electrostatic laws than do cellular networks; rather, these bioelectric networks are information rich networks which carry information in a bioelectric code which can be understood by the sender and receiver. (Levin 2014)
The Periodic Table of Elements
Now the electrostatic laws, in conjunction with the design of the periodic table of elements, constrain the possible chemical space of molecule bonding arrangements. For example, based on the chemical characteristics of hydrogen and oxygen as well as the electrostatic laws, H2O has a specific bonding configuration. These mechanisms can thus explain the origin and ready formation of some simple molecules. But what about more complex molecules like those used in life? According to a paper in the journal Nature, “Chemical space and biology,” “The chemical compounds used by biological systems represent a staggeringly small fraction of the total possible number of small carbon-based compounds with molecular masses in the same range as those of living systems (that is, less than about 500 daltons). Some estimates of this number are in excess of 1060.” (Dobson 2004) This statement is consistent with our observation that complex molecules like glucose and nucleic acids result from enzymes. If one thinks that electrostatic laws and the periodic table limit the search space so that molecules like nucleic acids form on their own, then nucleic acids should form spontaneously from phosphate, nitrogen, carbon, hydrogen, and oxygen, just like water does. But this is not something that is observed. Instead, complex molecules in an appreciable quantity can only be built using enzymes (which are built using information in DNA) or in highly controlled laboratory synthesis environments. Not to mention the fact that there must be something in the natural laws that “forces” the chemistry of life to use only left-handed molecules. And if that is true, then why aren’t all molecules left-handed as this would seem to require a “rule” in the laws.
If one grants the first cell (supposing the origin of life is a miraculous event), there remain thousands of unique molecular compounds essential for the diversity of life to be selected from the chemical space. We know that many of these molecular structures are multipurpose, recyclable, and essential to other ecosystem members. The design of these molecules and the enzymes that make and break them down appears to have required foresight for the needs and functions of the ecosystem as well as an in-depth understanding of chemistry and biochemistry. Is this type of information and causal power available in the electrostatic laws or the other laws of nature?
Laws of Thermodynamics
Definition: The first law of thermodynamics says that matter and energy cannot be created or destroyed but can only change form. The second law of thermodynamics says that closed systems always move toward states of greater disorder. Open systems move toward equilibrium, where the disorder (aka entropy) of the universe is at its maximum.
The laws of thermodynamics place constraints on what biological organisms must do to remain alive. That is, organisms must capture, harness, and expend energy to maintain a state far from equilibrium. To do this, organisms must/do have incredibly designed architectures that reflect a highly advanced understanding and exploitation of the laws of nature. For example, in central carbon metabolism, energy is extracted from the molecule glucose in the most efficient way possible. But just because this biochemical pathway exhibits an architecture that is amazingly designed to leverage the constraints imposed by thermodynamics does not mean that the laws provide a mechanism by which these complex systems arose in the first place. In other words, simply because a vehicle is highly efficient does not imply that the laws of thermodynamics designed it. More likely, it means whoever designed the vehicle had a thorough understanding of thermodynamics.
Quantum Physics
Definition: Quantum physics describes the physical properties at the level of atoms and subatomic particles using the wave function, which is determined by the Schrödinger equation. The Schrödinger equation is the quantum counterpart of Newton’s second law, describing what happens in the quantum realm to systems of subatomic particles.
Schrödinger equations are linear equations, so when added, the outcome is also linear. This is very different from what is observed in the real world. For biology and complex systems, conditional branching occurs, as in the example:
If {antibiotic is detected} then (express antibiotic efflux pump). If {antibiotic decreases} then (decrease expression of antibiotic efflux pump).
This type of branching found in complex systems cannot be boiled down to a wave function. Thus, as George Ellis, a leading theorist in cosmology and complex systems, says “[T]here is no single wave function for a living cell or macroscopic objects such as a cat or a brain.” In short, the complex nonlinear world is unable to arise from a single wavefunction.
Desire to Survive — NOT a Materialist Mechanism
Definition: The behavior that an organism programmatically/cognitively undertakes to avoid death.
The laws of physics and chemistry do NOT include natural selection. Natural selection is an outcome of the programming of a specific goal: desire to survive. As such, I define natural selection as the change in populations that depends upon their programmed and, in some cases, cognitive capacity to survive and the environmental factors they face. Please note that this definition is different from how most people might think of natural selection, but one hopes it is more accurately aligned with how it actually works. To support this goal, the “desire to survive,” organisms have a variety of mechanisms that may include both voluntary and involuntary responses. For example, in humans, the immune system would be an example of an involuntary response (programmatically compiled) where the defenses of the body fight off invaders. An example of a voluntary response (a cognitive response) in humans might be when someone runs for their life from a bear or kills a poisonous snake. Another example of an involuntary mechanism is natural genetic engineering. In case you aren’t familiar with natural genetic engineering, it just means that cells have the capability to actively reorganize and modify their own genomes to enable survival. This involves mechanisms like transposition (movements of genetic elements within the genome), gene duplication, horizontal gene transfer (transfer of genetic material between different organisms), and other forms of genetic rearrangement. Another important example is phenotypic plasticity, which has frequently been confused for natural selection but is the ability of an individual organism to exhibit different phenotypes (observable characteristics or traits), for example, in response to changes it senses in the environment. Phenotypic plasticity occurs too rapidly to be driven by mutation and selection; thus, it is recognized as an innate adaptation algorithm embedded within an organism.
So, the desire to survive, coupled with environmental conditions and random mutations that favor some individuals over others, is “natural selection.” As natural selection relies on the agent- or life-specific mechanism of a desire to survive, it cannot account for anything related to the origin of life, only the diversification of life. The degree to which natural selection can account for the diversification of life is an active area of research, but ID proponents Douglas Axe and Brian Miller have discovered some important limits. Miller summarized decades of research on the topic of protein evolution, which relies on natural selection, in our response to Rope Kojonen. In short, they have shown that natural selection is not capable of creating a high-complexity enzyme from a random sequence of amino acids or of transforming one protein fold into a different fold without guidance. This is effectively an upper bound for what natural selection can accomplish, which bears not only on origin-of-life scenarios but also on the ability of life to diversify from a single organism into the diversity we see today.
Necessary but Not Sufficient
The emergent properties of physics and chemistry are necessary, but not sufficient to explain the origin or diversification of biological organisms. Gravity can be used as a cue by biology to determine directionality, but gravity doesn’t make a leaf grow up or a root grow down — that happens only because a complex system is sensing, interpreting, and acting on the gravitational cue. The design of the periodic table of elements constrains the bonding pattern between hydrogen and oxygen and bestows upon water its life-giving properties, but these constraints on chemical bonding do not cause the formation of DNA or other complex molecules. Enzymes are necessary for more complex molecules to be formed at the rate required for life. The electrostatic laws describe how positive and negative charges attract one another, but these laws do not cause the formation of an electrochemical gradient across a membrane — that only happens because molecular machines harness energy to push a system away from equilibrium. In quantum physics, the linear wave function describes the wave-particle duality of matter, but it cannot account for the conditional branching observed in complex systems.
In short, the best way to summarize the capacity of all these material mechanisms is in George Ellis’s words from his recent article, “Quantum physics and biology: the local wavefunction approach”: “The laws of physics do not determine any specific outcomes whatsoever. Rather they determine the possibility space within which such outcomes can be designed.” (Ellis 2023)
Tomorrow we will look at the second interpretation of Kojonen’s model for how the laws of nature and initial conditions could bring about life and its diversification.